Cell Cycle Series: History and Discovery
Foreword
Have you ever questioned how a wound heals? How does an embryo become a baby? How does a cancerous tumor grow? At the heart of these questions lies cell replication and division. The remarkable ability of a cell to grow and divide into two identical daughter cells underpins the success of life. This is, however, only conceivable because eukaryotic cells (that is, with nuclei) go through the cell cycle, a set of well-defined and tightly synchronized events. This process is divided in two main stages: the interphase, whereby a cell grows; and the mitotic phase, by which cells replicate (mitosis) and divide (cytokinesis). During this process deoxyribonucleic acid (DNA), also recognized as the "molecule of life," is accurately replicated and faithfully distributed to newly generated daughter cells. DNA is the molecule that carries and conveys hereditary material or genetic information from parents to offspring, thus holding all the information needed to construct and sustain an organism. The cell cycle thus functions as the master regulator of tissue growth, development and proliferation. It also ensures that old cells are removed in a timely manner as we age and replaced by new cells that proliferate in a tightly controlled fashion. This keeps the total number of viable cells constant, which is essential for life as we know it.
The Cell Cycle Series attempts to elucidate the most important aspects of the cell cycle: from its history and discovery to the underlying mechanisms that control cell division. This series aims to explore the intricacies of cell cycle control in development and the consequences of its failure in disease.
Therefore, this series is divided into the following chapters:
1. Cell Cycle Series: History and Discovery
Cell Cycle Series: History and Discovery
By the means of Telescopes, there is nothing so far distant but may be represented to our view; and by the help of Microscopes, there is nothing so small as to escape our inquiry; hence there is a new visible World discovered to the understanding.
Robert Hooke, 1665

While the telescope opened up the universe to human gaze, the microscope unleashed smaller worlds, revealing what living forms were made of. What do bacteria, a poppy, a dog and a human have in common? Regardless of their external appearance, they all share important characteristics, such as the ability to adapt and respond to environmental stimuli, process energy, maintain homeostasis (to balance the internal environment), reproduce, and grow. According to the cell theory, which was established by Schleiden and Schwann in 1839 (Schleiden, 1838; Schwann, 1839) and has since remained the cornerstone of contemporary biology, these traits all converge in the basic building unit of life from which all organisms are made: the cell.
The Architecture of a Cell: Concept to design
Before delving into the steps a cell must take to replicate, one must first understand its structure and function, as well as its genetic information. Despite the fact that the human body is made up of hundreds of distinct cell types, each with a unique form, size, and function, all cells have a conserved structure. Aside from prokaryotic cells, which lack a nucleus and are fundamentally linked to single-celled organisms; eukaryotic cells, which compose multicellular organisms, share a structural hallmark known as compartmentalization (Vellai, 1999). The link between structure and function is clear in nature at all levels, including at the cellular level: eukaryotic cells are the epitome of the "form follows function" principle. Eukaryotic cells have an organized nucleus and organelles (compartments) enclosed by a membrane, with each organelle neatly separated from one another by layers of membrane. This structural organization ensures that different compartments have unique functions while maintaining the necessary conditions for each compartment's specific purpose (Alberts, 2002).
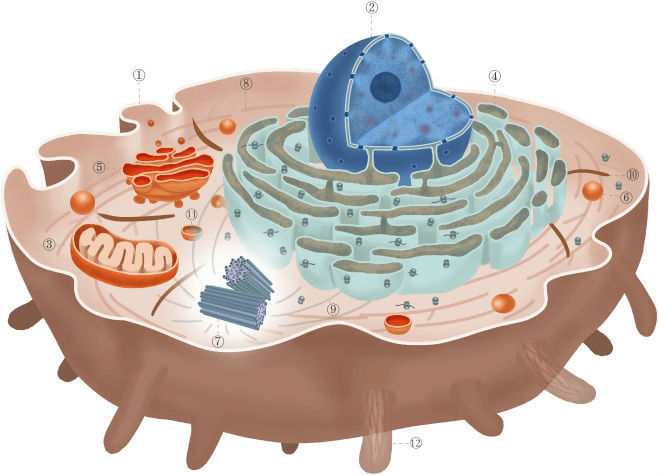
Cells are the basic components of life in part because they exist in separate and clearly identifiable bundles. Each cell is enveloped by a cell membrane (also referred to as plasma membrane), which acts as a clear barrier between the cell's internal and external environments. Within the cell membrane lies the cytoplasm, where most cellular activities take place and most of the cellular organelles are located - apart from the nucleus. The nucleus is one of the most important eukaryotic organelles. In fact, the presence of a nucleus is considered one of the distinguishing features of an eukaryotic cell. The nucleus is a double-membraned organelle (both membranes forming the nuclear envelope) which is vital for every cell (and organism) not only because it houses the cell's genetic material in the form of chromosomes, i.e., tightly coiled structures consisting of protein and a single molecule of DNA that transmits genetic information from cell to cell, but also because it serves as the site where the DNA is first interpreted (Alberts, 2002).
From Monasteries to the Cell
While the principles of magnification and optics are centuries old, wearable solid glass lenses were not engineered until the Middle Ages (Cashell, 1971). Suitable microscopes for solid scientific purposes, however, were not available until the 17th century (Wollman, 2015). It was not until 1665 that the term "cell" was first coined by Robert Hooke, a pioneering scientist who improved the design of primitive light microscopes to better capture the world of what was invisible to the human eye (Hooke, 1665). Micrographia, Hooke's masterpiece, is the first book on microscopy written by a scientist that faithfully describes a hitherto unexplored microscopic world (Hooke, 1665). It contains not only countless scientific hypotheses on various subjects, but also descriptions of biological and other specimens, supplemented by Hooke's magnificent original drawings. By carefully examining samples of cork under a microscope, Hooke identified cellula-like structures (from the Latin word cellula, meaning monk's chamber) resembling small rooms in which monks resided - hence originating the term cell (Hooke, 1665). Unbeknown to Hooke, the cork cells had long ago died and therefore lacked the internal structures typically seen in living cells. While Hooke's observations provided no evidence of the nucleus or other organelles found in most living cells; Hooke made significant advances in the microscopy field, the backbone of microbiology, by, along with Antoni van Leeuwenhoek, establishing the existence of microscopic organisms (Gest, 2004).
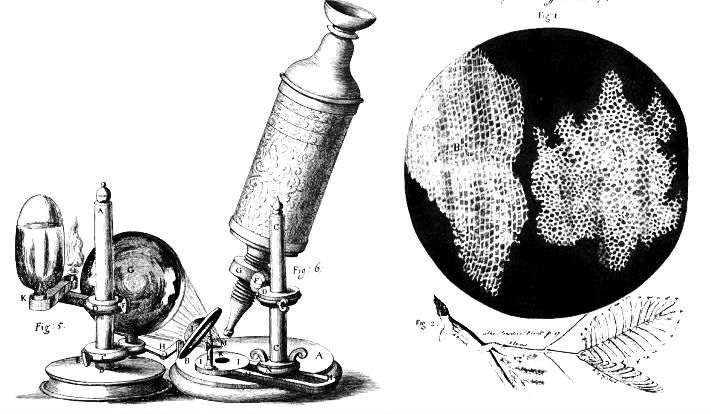
Cell Theory: A Unifying Concept
Hooke’s significant contributions prompted in-depth microscopic studies of several living biological species. However, many scientists refused to use early microscopes because they were skeptical of what they observed. Aberrations and imperfections in the lenses often led to image distortions, resulting in misleading observations (Lister, 1830; Mazzarello, 1999). Over the following 200 years, the basic microscope design remained largely unchanged, but advances in lens manufacture (including the use of higher purity glass) helped overcome difficulties such as blurriness and poor image resolution. Compound microscopes have been fitted with mirrors to increase light and improve image quality. One of the first microscopes tackling the earlier challenges of less refined microscopes was created by Joseph Jackson Lister in conjunction with instrument craftsman William Tulley (Lister, 1830). The discovery of an entire world of microscopic creatures sparked a boom among the most inquisitive of scientists and was seen as a visible link between inanimate matter and living organisms (Mayr, 1982).
Advancements in microscope design, staining methods, and lens technology enabled scientists to identify specific components within cells. This paved the way for the zoologist Theodor Schwann and the botanist Matthias Schleiden, who studied cells in animals and plants respectively, to construct the classical cell theory. Schleiden and Schwann's names are just as tightly connected with the origins of cell theory as Watson and Crick's are with DNA. While in 1838 Schleiden first hypothesized that cells are the structural element of plants (Schleiden, 1838), the following year Schwann expanded on Schleiden's core ideas by suggesting that all tissues, including those of plants and animals, consist of cells (Schwann, 1839). This set the groundwork for the idea that not only is the cell the structural basis of all living things, but all living things are made up of cells, i.e., cells are the building blocks of life. Schleiden later advocated that cells spontaneously arise by crystallization of intercellular non-living matter, also referred to as granules (Mayr, 1982; Schwann, 1843). Collectively, these hypotheses laid the foundation for the cell theory. Despite being erroneous, spontaneous generation, which is the hypothetical process through which living organisms evolve from inanimate matter, was a long-held and prevailing belief at the time. Interestingly, Schwann was the first to present evidence that contradicted spontaneous generation. Schwann discovered that when nutritional broth was boiled and sterilized, no microorganisms could grow and no biological nor chemical activity could be detected. This experiment led Schwann to believe that all microorganisms had been destroyed and that no more could be formed, indicating that the spontaneous generation hypothesis was likely incorrect (Locy, 2016).

It was only twenty years later that the spontaneous generation theory was abandoned in favor of a doctrine supporting that each cell descended from a pre-existing one. In 1855, Rudolph Virchow refuted spontaneous generation with his now-famous Latin aphorism "omnis cellula e cellula", meaning all cells come from cells (Virchow, 1863; Wright & Poulsom, 2012). The inability to prove spontaneous creation led Virchow to propose the theory of biogenesis, suggesting that just as animals are unable to arise without previously existing animals, cells are unable to arise without previously existing cells. However, numerous scientists questioned Virchow's idea since it lacked substantial scientific support (Kearl, 2012). Despite lacking solid foundations, this discovery served as the catalyst for cell cycle research.
Since the development of cell theory, impressive breakthroughs and advances with microscopes have paved the way for new discoveries, culminating in the modern cell theory. This theory postulates that all cells have a similar chemical composition, with metabolites flowing through them and that genetic material, the blueprint for life, is transferred to daughter cells during cell division. This is still the prevailing doctrine nowadays and the foundation of all living things (Mazzarello, 1999; Ribatti, 2018).
The Birth of a Cell: From Matter to Cell Division
Even though images of dividing cells had been observed virtually since the invention of the microscope, it took time for scientists to recognize that such images actually represented the process of cell formation. The genesis of cells was not a concern among the scientific community until the early eighteenth century, and it took considerably longer for scientists to acknowledge that division was the mechanism underlying cell formation. The work of Carl Nägeli and Robert Remak in the 1850s, who precisely outlined the division of plant and animal cells, and Virchow, who reaffirmed the idea that all cells are generated from a previously existing one, provided a comprehensive picture of the cell division process (Harris, 1999). However, despite the avalanche of advances in microscopic methods and instruments in the 19th century, most microscopic studies of cells were undermined by the fact that cellular internal components were either translucent or transparent, rendering it impossible to characterize their functions and structural features. In an unwavering effort to overcome this, scientists developed staining methods to dye cells and hopefully highlight key cellular components. Most dyes, however, were toxic and lethal to cells, or tinted the entire cell a dark hue, therefore masking the hidden structures they were intended to reveal (Alberts, 2002).

Pioneering the use of newly identified aniline dyes to visualize cell structures, Walther Flemming discovered that a particular class of dyes intercalated exceptionally well with threadlike elements in the cell nucleus (Flemming, 1882). These elements were first named “chromatin", the building block of chromosomes, consisting of DNA and protein. Chromosomes (meaning colored bodies), however, consist of tightly coiled DNA that carries genomic information from cell to cell through the basic physical and functional unit of heredity: genes. Flemming applied these dyes to cells killed at distinct phases of division and prepared a sequence of slides that, when examined under a microscope clearly pinpointed the changes occurring in the cell nucleus during mitosis. Flemming unveiled the intricacies of chromosomal movement during mitosis, revealing how chromosomal fibers arise from the nucleus, which then split longitudinally before shortening and thickening later in mitosis (Flemming, 1965; Huxley, 1925). Flemming observed a pattern in which cells cycle from a resting state to a time of frenetic activity, culminating in the conversion of one nucleus into two, followed by a stage in which the cell is torn apart to further divide into two identical cells. Each of these cells contains its own chromatin components entirely wrapped within the nucleus. Flemming’s findings were later corroborated by the study of live dividing cells (Paweletz, 2001). In the modern age, the cyclic process of a nucleus dividing into two is known as mitosis, and the cell partitioning itself is known as cytokinesis. These two processes combine to form essential components of the cell cycle (O'Connor, 2008).
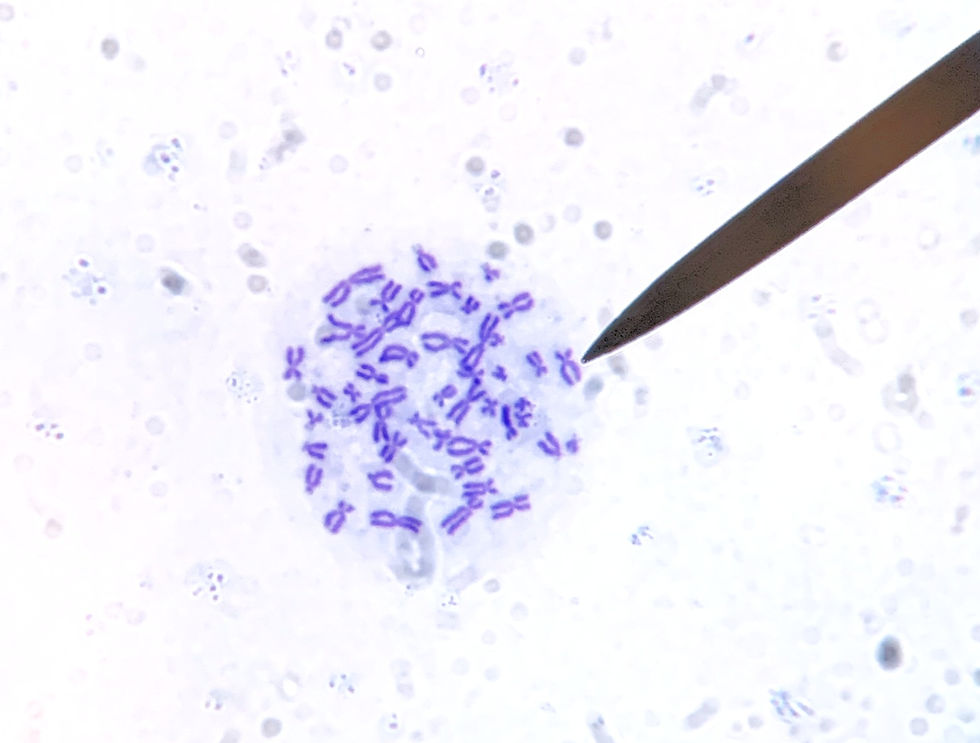
The Life of a Cell: Replication and Division
The study of the cell cycle experienced a sort of Dark Age between 1920 and 1950. Most research has been limited to studying possible chromosomal abnormalities that can occur during mitosis and the implications for genetic transmission (Darlington, 1958). In 1953, the discovery of the double helix, the twisted ladder structure of deoxyribonucleic acid (DNA), the molecule of life, which contains the genetic information responsible for the development and function of an organism, by James Watson and Francis Crick was a turning point in science (Watson & Crick, 1953), providing insight into the genetic code and protein synthesis, laying the foundation for the development of new and powerful scientific techniques in the 1970s and 1980s (Durmaz, 2015; Mornet, 2003).
That same year, however, a largely forgotten study, pioneered by Alma Howard and Stephen R. Pelc on cell proliferation in bean roots, paved the way for the discovery of the cell cycle model as we know it today (Howard & Pelc, 1953). Howard and Pelc were the inventors of autoradiography and the first to successfully assign a time frame to a cell's life, i.e., to discover the cell cycle. Using a botanical experimental system, the bean root Vicia faba, they observed that DNA replication only takes place at a particular phase of the cell cycle, clearly distinct from mitosis (Howard & Pelc, 1951, 1953). They named this stage S-phase from DNA synthesis, now recognized as interphase, during which the cell grows, duplicates its DNA and prepares for mitosis. This paved the way for further identification of the four characteristic phases of the cell cycle: mitosis (M), gap 1 (G1), DNA synthesis (S), and gap 2 (G2). The concept of the cell cycle was born.
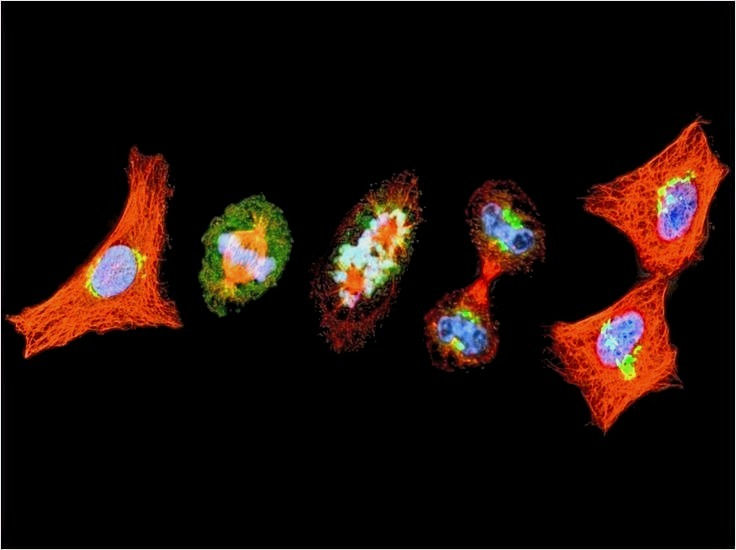
Conclusions
The microscope revealed an amazing new world, a universe of cellular life. The discovery of cells had a far greater impact on science than Hooke could have foreseen in 1665: it not only provided us with fundamental knowledge about the building blocks of all living species, but also led to improvements in medical technology and therapy. Technology has advanced since the birth of classical cell theory, enabling broader observations that have led to new discoveries about cells, particularly the emergence of modern cell theory, which is the most important cornerstone of contemporary biology. The cell concept or cell theory states that all life is made up of cells. That is, the cell is the basic unit of life and the simplest unit that exhibits the characteristics of life.
From the smallest creatures consisting of a single cell, including many kinds of bacteria and parasites, to the sophisticated human body consisting of trillions of cells interacting with one another, we are all founded on the cell. From the moment we are first conceived, we are constantly evolving and growing: this owes to the cell's life cycle and to the extraordinary ability of one cell in becoming two. Have you ever seen a caterpillar transform into a butterfly? If so, you are probably familiar with the concept of a life cycle. Butterflies go through some pretty impressive life cycle transformations, from worms to chrysalis to majestic and colorfully winged creatures. Other organisms, from humans to plants to microbes, all have a life cycle: a sequence of developmental processes that each individual goes through from birth to reproduction. The cell cycle can be understood as the life cycle of a cell. In other words, it is the sequence of developmental and growth processes that a cell undergoes between birth, through the division of a mother cell, and reproduction, to produce two new daughter cells. The modern concept of the cell cycle is founded on Howard and Pelcs' findings and was further refined using genetic and molecular biology techniques, which revealed the biochemical and molecular events occurring at each stage of a cell's life. They also shed light on the underlying mechanisms that govern cell transitions through the many phases of the cell cycle. In modern biology, these systems are known as cell cycle checkpoints, and they will be discussed in greater depth later in this series.
Bibliographical References:
Alberts, B., Johnson, A., & Lewis, J. (2002). An Overview of the Cell Cycle. In Molecular Biology of the Cell (4th ed.). Garland Science.
Cashell G. T. (1971). A short history of spectacles. Proceedings of the Royal Society of Medicine, 64(10), 1063–1064.Darlington, C. D. (1958). Evolution of genetic systems. Cambridge University Press. https://books.google.es/books?id=UuHcwAEACAAJ
Darlington, C D. 1958. Evolution of Genetic Systems. Oliver and Boyd, Edinburgh.
Durmaz, A. A., Karaca, E., Demkow, U., Toruner, G., Schoumans, J., & Cogulu, O. (2015). Evolution of genetic techniques: past, present, and beyond. BioMed research international, 2015, 461524. https://doi.org/10.1155/2015/461524
Flemming, W. (1882). Zellsubstanz, Kern und Zelltheilung. F.C.W. Vogel. /https://doi.org/10.5962/bhl.title.168645
Flemming, W. (1965). Contributions to the knowledge of the cell and its vital processes. Part II. Journal of Cell Biology, 25(1), 3–69. https://doi.org/10.1083/jcb.25.1.3
Gest H. (2004). The discovery of microorganisms by Robert Hooke and Antoni Van Leeuwenhoek, fellows of the Royal Society. Notes and records of the Royal Society of London, 58(2), 187–201. https://doi.org/10.1098/rsnr.2004.0055
Harris, H. (1999). The Birth of the Cell. In Journal of the History of Biology (pp. 98–104). Yale University Press.
Hooke, R. (1665). Micrographia, or, Some physiological descriptions of minute bodies made by magnifying glasses :with observations and inquiries thereupon. Printed by Jo. Martyn and Ja. Allestry, printers to the Royal Society. https://doi.org/10.5962/bhl.title.904
Howard, A., & Pelc, S. R. (1951). Synthesis of nucleoprotein in bean root cells. Nature, 167(4250), 599-600. https://doi.org/https://doi.org/10.1038/167599a0
Howard, A., & Pelc, S. R. (1953). Synthesis of Deoxyribonucleic Acid in Normal and Irradiated Cells and Its Relation to Chromosome Breakage. Heredity, 6, 261–273. https://doi.org/https://doi.org/10.1080/09553008514552501
Huxley, J. S. (1925). The Cell in Development and Heredity. Nature, 115(2897), 669–671. https://doi.org/10.1038/115669a0
Kearl, Megan, "Rudolf Carl Virchow (1821-1902)". Embryo Project Encyclopedia (2012-03-17). ISSN: 1940-5030 http://embryo.asu.edu/handle/10776/2274.
Lister, J. J. (1830). On some properties in achromatic object-glasses applicable to the improvement of the microscope. Philosophical Transactions of the Royal Society of London, 120, 187–200. https://doi.org/10.1098/rstl.1830.0015
Loci, W. (2016). Biology and Its Makers. WENTWORTH Press. https://books.google.es/books?id=JPJdvgAACAAJ
Mayr, E. (1982). The Growth of Biological Thought. In American Anthropologist. The Belknap Press of Harvard University Press Cambridge. https://doi.org/10.1525/aa.1986.88.2.02a00160
Mazzarello, P. (1999). A unifying concept: The history of cell theory. Nature Cell Biology, 1(1), E13–E15. https://doi.org/10.1038/8964
Mornet E. (2003). ADN, les 50 ans de la double hélice : du concept d'hybridation moléculaire aux biopuces [DNA, 50 years of the double helix: from the concept of molecular hybridization to microarrays]. Gynecologie, obstetrique & fertilite, 31(11), 895–899. https://doi.org/10.1016/j.gyobfe.2003.06.001
O'Connor, C. (2008) Cell Division: Stages of Mitosis. Nature Education1(1):188
Paweletz, N. (2001). Walther Flemming: Pioneer of mitosis research. Nature Reviews Molecular Cell Biology, 2(1), 72–75. https://doi.org/10.1038/35048077
Pelc, S. R. (1947). Autoradiograph technique. Nature, 160(4074), 749. https://doi.org/https://doi.org/10.1038/160749b0
Ribatti, D. (2018). An historical note on the cell theory. Experimental Cell Research, ,364(1). https://doi.org/https://doi.org/10.1016/j.yexcr.2018.01.038
Schleiden, M. J. (1838). Beiträge zur Phytogenesis. In J. Müller (Ed.), Müllers Arch. Anat. Physiol. Archiv für Anatomie, Physiologie und Wissenschaftliche Medicin. https://lccn.loc.gov/sf85004110
Schwann, T. (1839). Mikroskopische Untersuchungen über die Uebereinstimmung in der Struktur und dem Wachsthum der Thiere und Pflanzen (G. E. Reimer (ed.)). Sander. https://books.google.co.ao/books?id=m9ZcAAAAcAAJ
Schwann, T., & Smithe, H. (1843). Microscopical researches into the accordance in the structure and growth of animals and plantas. Printed for the Sydenham Society.
Sharp, L. W. (1921). An introduction to cytology. McGraw-Hill book company, inc. https://doi.org/10.5962/bhl.title.921
Vellai, T., & Vida, G. (1999). The origin of eukaryotes: the difference between prokaryotic and eukaryotic cells. Proceedings. Biological sciences, 266(1428), 1571–1577. https://doi.org/10.1098/rspb.1999.0817
Virchow, R. (1863). Cellular pathology as based upon physiological and pathological histology. In F. Chance (Ed.), Pathological Insitute of Berlin (2nd ed.). J. B. Lippincott. https://doi.org/10.5962/bhl.title.32770
WATSON, J., CRICK, F. Molecular Structure of Nucleic Acids: A Structure for Deoxyribose Nucleic Acid. Nature 171, 737–738 (1953). https://doi.org/10.1038/171737a0
Wollman, A. J., Nudd, R., Hedlund, E. G., & Leake, M. C. (2015). From Animaculum to single molecules: 300 years of the light microscope. Open biology, 5(4), 150019. https://doi.org/10.1098/rsob.150019
Wright, N. A., & Poulsom, R. (2012). Omnis cellula e cellula revisited: Cell biology as the foundation of pathology. Journal of Pathology, 226(2), 145–147. https://doi.org/10.1002/path.3030
Visual Sources:
Figure 1: Montgomery, R. (2013). Microscope or Telescope Drawing. Talenthouse. [image] https://www.talenthouse.com/richiemontgomery
Figure 2: Zhao, W., Ren, W., Huang, D., Sang, Y., Cao, L., & Huang, J. (2022). Chapter 1 - Cell structure and physiology. In M. Schnoor, L.-M. Yin, & S. X. Sun (Eds.), Cell Movement in Health and Disease (pp. 3–16). Academic Press. https://doi.org/https://doi.org/10.1016/B978-0-323-90195-6.00007-3
Figure 3: Hooke, R. (1665). Micrographia, or, Some physiological descriptions of minute bodies made by magnifying glasses: with observations and inquiries thereupon. Printed by Jo. Martyn and Ja. Allestry, printers to the Royal Society. https://doi.org/10.5962/bhl.title.904. [image]
Figure 4: Lubczynski, J. Cell Theory Timeline. (n.d.) [image]. Sutori. https://www.sutori.com/en/story/cell-theory-timeline--Q4t9g4MpHS5GdVxgFS8fojUA
Figure 5: Rieder, C. L., & Khodjakov, A. (2003). Mitosis Through the Microscope: Advances in Seeing Inside Live Dividing Cells. Science, 300 (5616), 91–96. https://doi.org/10.1126/science.1082177
Figure 6: ScienceAlert. [@ScienceAlert]. (2021, October 11). A single DNA molecule makes up each delicate X shapes magnified 2000x. [Image]. Twitter. https://twitter.com/DNA_RNA_Uni/status/1429136569638825991?s=20&t=R5LMk05s90s2_NrXZQf8aA
Figure 7: Cultured Cells. (2012) [image]. National Center for Microscopy and Imaging Research (NCMRI). https://3dem.ucsd.edu/gallery/index.shtm
Opmerkingen