Epidemiology of Infectious Diseases: The Chain of Infection and Transmission Dynamics
The classic epidemiological triad model holds that infectious diseases result from the interplay of agent, host, and environment. For each infectious disease, however, such a relationship demands a specific connected series of events referred to as the chain of infection or chain of transmission. The chain starts with an infectious agent dwelling and proliferating in a natural reservoir that supports the infectious agent's survival in nature, such as a human, animal, or the environment (soil or water). The infectious agent exits the reservoir through an exit portal and travels through some route of transmission to a portal of entry into a vulnerable host (Ryu et al., 2022). An in-depth understanding of the chain of infection is critical for disease management and prevention in the context of infectious diseases, as interrupting a link within the chain will stop the infectious agent from spreading. More than one intervention can sometimes be beneficial in controlling disease spread, and the method selected can be determined by a range of factors such as economics and the ease with which an intervention may be implemented in a specific setting (van Seventer & Hochberg, 2017). It is important to acknowledge that the potential for rapid and extensive spread of infectious organisms as a consequence of globalization demands coordinated efforts within and across states to guarantee successful disease prevention and control.
"Typhoid Mary": The Infectious Agent and Its Reservoir
Every infectious disease originates from an infectious agent that lives, thrives and multiplies in a reservoir. Humans, animals, and the environment all serve as potential disease reservoirs that are often, but not always, the source from which the pathogen is transmitted to a vulnerable host. For instance, while the bacterium Clostridium botulinum (C. botulinum) which causes botulism, is found in soil, improperly stored food that carries C. botulinum spores is the main contributor to disease transmission to humans (Peck, 2014). Besides the environment, also animals serve as a reservoir for a significant number of human diseases through zoonosis, which account for about 60% of all infectious diseases (Burke et al., 2012). One such example is the Marburg virus, which, like Ebola, causes hemorrhagic fevers with extremely high mortality rates and whose natural reservoir are bats. Bats transmit the virus to both humans and other wildlife such as African gorillas. Although the infection in these wild animals is too severe and therefore they cannot serve as a reservoir for the virus in nature (since they would die too early), they may still transmit Marburg viruses to humans (“In the News,” 2009). Measles, mumps, streptococcal infections, smallpox, HIV, and respiratory illnesses like influenza, on the other hand, are only a few of the prevalent infectious diseases for which human serve as reservoirs (Principles of Epidemiology | Lesson 1 - Section 10, 2012; Tulchinsky & Varavikova, 2014). Notable is the successful example of smallpox eradication, which was only possible since humans were the only reservoir of the smallpox virus. Once the last human case was identified and isolated, naturally occurring smallpox was thereby eradicated.
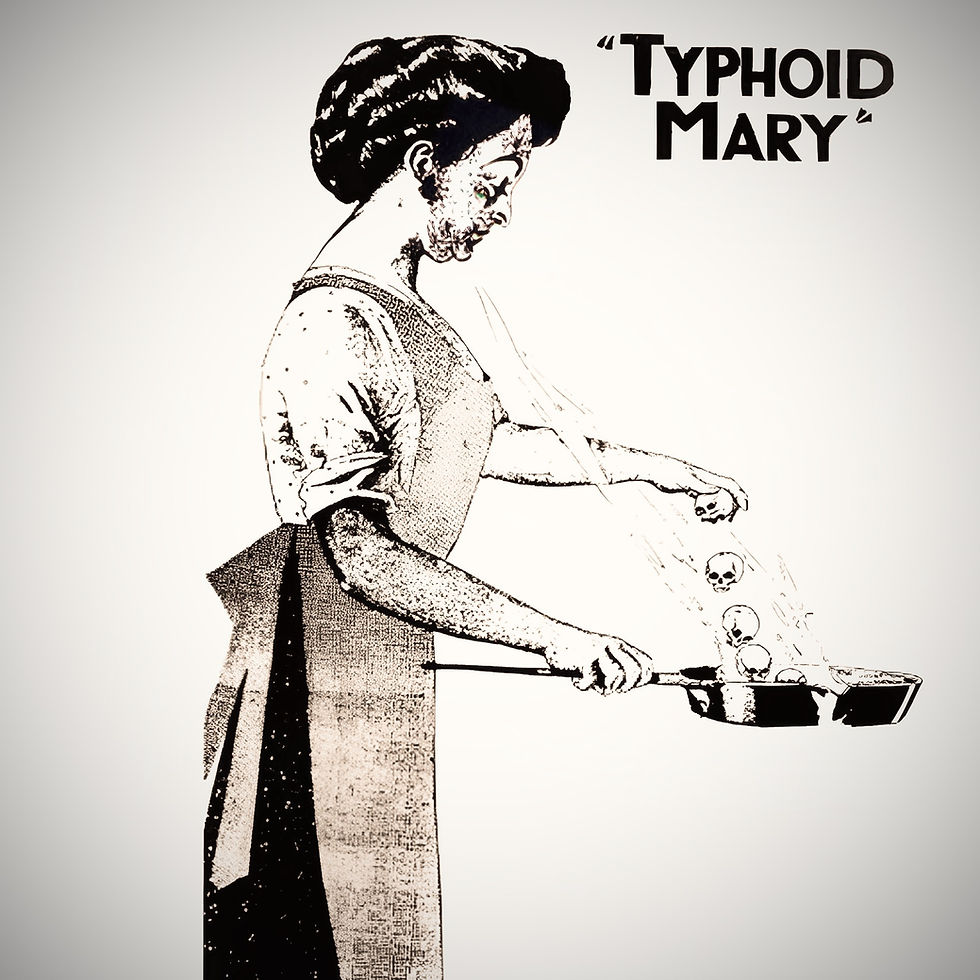
From a public health standpoint, one essential feature of human reservoirs is that they might not exhibit symptoms of disease and so may serve as undetected disease carriers (and spreaders) within communities. Cook Mary Mallon, also known as “Typhoid Mary”, is a prime illustration of a human reservoir. In 1906, a Long Island landlord was trying to track down the cause of a typhoid outbreak after renting his property to a family of bankers and servants for the summer. By the end of August, six of the house's eleven residents were suffering from typhoid fever. George Sober, a civil engineer by training who had become an expert on sanitation, was tasked with solving this mystery. A self-described “epidemic fighter”, Soper believed that a single person could spread the disease by acting as a carrier, i.e. without showing symptoms. He focused his attention on Mary Mallon, the Long Island's cook who had arrived three weeks before the first person fell ill (Strochlic, 2020). While he first assumed soft clams prepared by Mary were the culprits, this proved incorrect because not everyone affected with the disease consumed them. By ascertaining that the cook had previously served in eight homes, seven of which contracted typhoid disease, Sober ultimately found the answer to the puzzle. “Typhoid Mary” was an asymptomatic chronic carrier of Salmonella typhi, the bacterium responsible for typhoid fever, and has been linked to at least 53 instances of typhoid fever (Leavitt, 1996). She spent more than a third of her life in isolation alongside other inmates. Since so many people have contracted the disease as a result of her denial of having it, she has come to symbolize the disease's spread (Marineli et al., 2013).
Ready For Takeoff: Portal of Exit and Transmission Routes
A pathogen departs its reservoir through a portal of exit, which often reflects the primary site of the disease. As an illustration, respiratory pathogens such as influenza virus typically exit the body through respiratory secretions, whereas gastrointestinal pathogens such as rotavirus and Cryptosporidium spp. typically leave the body via fecal sources (Louten, 2016). For human reservoirs, sites where the host's blood, breast milk, sperm, and urine exit also serve as exit ports. It is important to note that different exit portals allow the same infectious agent to leave its reservoir. For instance, while SARS-CoV-2 predominantly left human hosts through respiratory secretions, a significant outbreak in China was reported to have started as a result of the virus spreading through fecal sources (Yu et al., 2004).
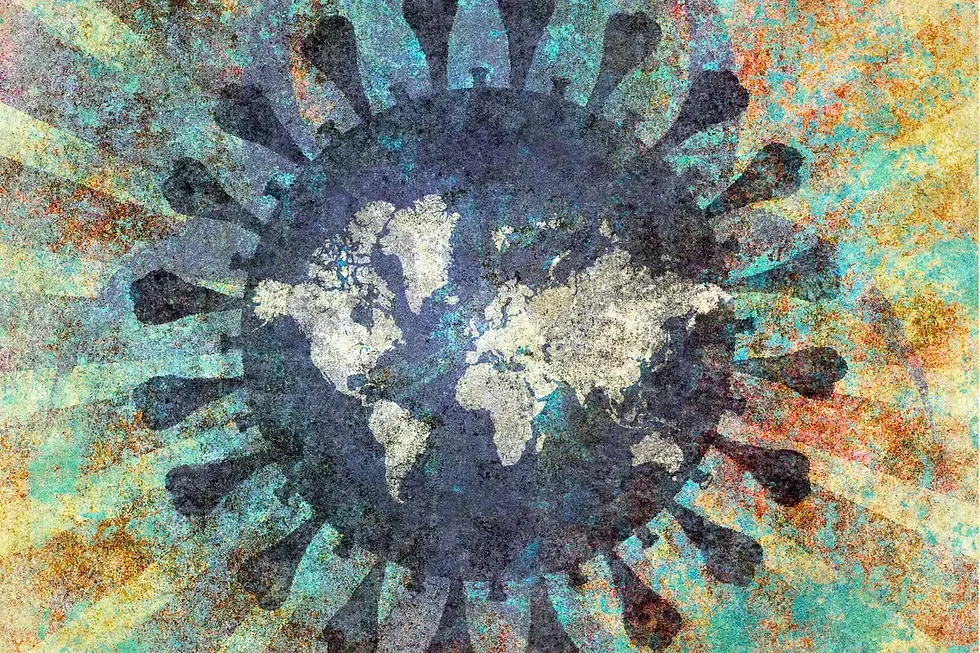
There are several possible transmission modes, or ways through which an infectious agent might spread from the natural reservoir, or the portal of escape, to a vulnerable host. Infectious disease transmission is often categorized in direct transmission, when the infective agent is conveyed directly from a reservoir to the host, and indirect transmission, which occurs when the agent is conveyed to the final host via a living or inanimate intermediary. Direct contact or droplet spreading during direct transmission are the two methods used to transmit an infectious agent from a reservoir to a susceptible host (Ryu et al., 2022). Skin-to-skin contact, kissing, and sexual contact are all examples of direct contact, as is coming into touch with soil or plants that have been infected with an infectious organism. Contrarily, droplet spread refers to the spray of relatively large, short-range aerosols brought on by talking, sneezing, or even coughing (Louten, 2016). Although it may be questioned whether this actually constitutes indirect transmission, droplet spread is categorized as direct since dissemination occurs by direct spray over a short distance before the droplets reach the ground. Meningococcal infection and pertussis (whooping cough) are two diseases that can spread through droplet dissemination from an infected patient to a vulnerable host (Farnsworth, 2022; Shaklee, 2012). An infectious agent can be transmitted indirectly from a reservoir to a host through airborne particles, inanimate objects (vehicles), or living intermediates (vectors). Airborne transmission occurs when bacteria or viruses migrate in droplet nuclei that become aerosols. Such droplets are formed when the liquid from pathogenic droplets produced by coughing or sneezing evaporates and can remain airborne for a long period of time and spread over considerable distances (Wang et al., 2021).
Vehicles can spread an infectious agent indirectly by passively transporting it - similar to how food and water can transmit the hepatitis A virus—or by creating an environment in which the infectious agent can grow, multiply, or produce a toxin—such as the aforementioned improperly canned food which provides an environment that supports the production of botulinum toxin by Clostridium botulinum (Peck, 2014; Sattar et al., 2000). Vehicles, therefore, include food, water, biological products (blood), and fomites, the latter including any inanimate objects on which infectious agents can survive and which serve as a vector for human-to-human transmission. Finally, vectors such as mosquitoes, fleas, and ticks can transmit an infectious agent in a purely mechanical manner, or promote the growth or changes of the agent. Examples of mechanical transmission include fleas carrying Yersinia pestis, the causative agent of the plague. Another illustration of interest, is the malaria pathogen which matures in an intermediate host, the mosquito vector, before it can be transmitted to humans (Hinnebusch & Erickson, 2008; Jr. et al., 1991).
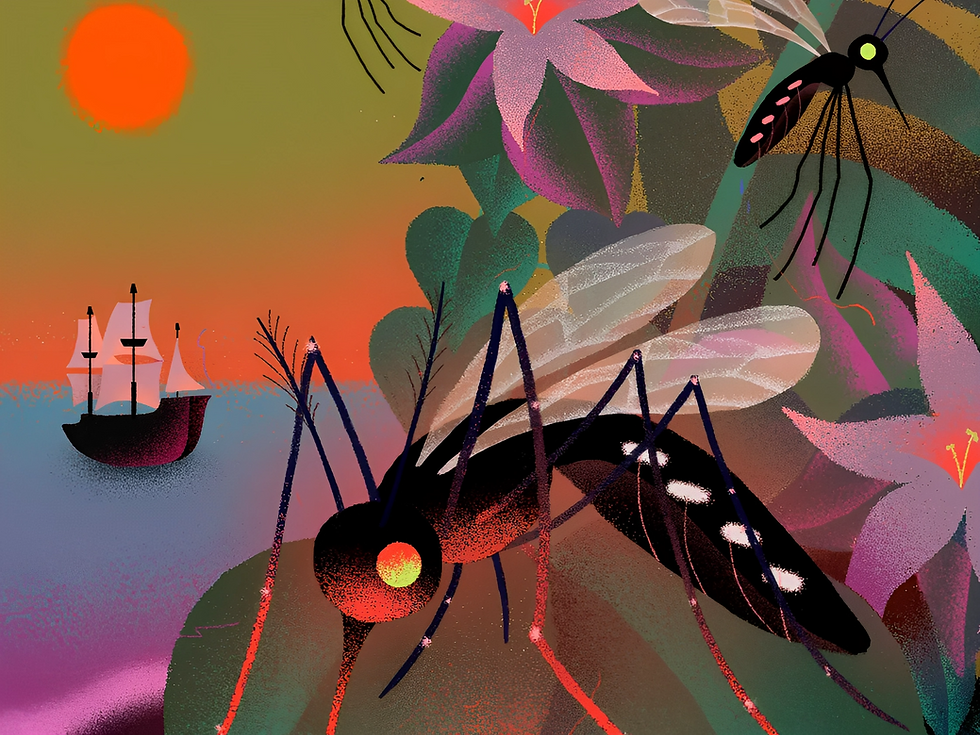
R-Naught: Understanding Transmission Predictions
An essential piece of the jigsaw epidemiologists strive to put together when analyzing an epidemic is how quickly a novel virus spreads from one case to multiple cases. The basic reproductive number, R0 (pronounced R naught), is the epidemiologists' go-to approach, and forecasting and projection are key research concerns in each pandemic. The R0 reflects the average number of individuals to whom a single infected individual is likely to spread the disease. In other words, it is an estimate of an infectious disease's average potential to spread (Ramirez, 2023). R0 offers crucial information regarding the possible spread of a disease that local, state, and federal governments, as well as public health organizations, may take into account when deciding how to manage a disease outbreak. Consequently, this parameter has been used to characterize the contagiousness or transmissibility of infectious agents associated with various communicable diseases that have affected mankind throughout history (Delamater et al., 2019). Furthermore, because it can be calculated for many diseases, it enables one to contextualize an epidemic with ones that have already been reported.
For instance, if the R0 for a disease in a susceptible population is 5, it means that on average, five susceptible hosts will get the disease from one infected case. If the R0 is more than one, transmission is anticipated to continue in a community; however, if the R0 is less than one, transmission is projected to tail off since, on average, less than one person is infected by a single infected individual (Yadav et al., 2021). This number is the outcome of a sophisticated modeling procedure that generates estimations based on numerous sources of information. The disease’s infectious period, or how long an infected person remains contagious; the mode of transmission and the contact rate, i.e., a gauge of how many people an infected person is likely to come into contact with, are three essential factors that must be taken into account when determining R0. Unlike the first two, the latter factor is not disease-specific. Instead, it is impacted by a variety of variables, including local and public health interventions like quarantines or travel restrictions (Gunderson & Woskie, 2020).
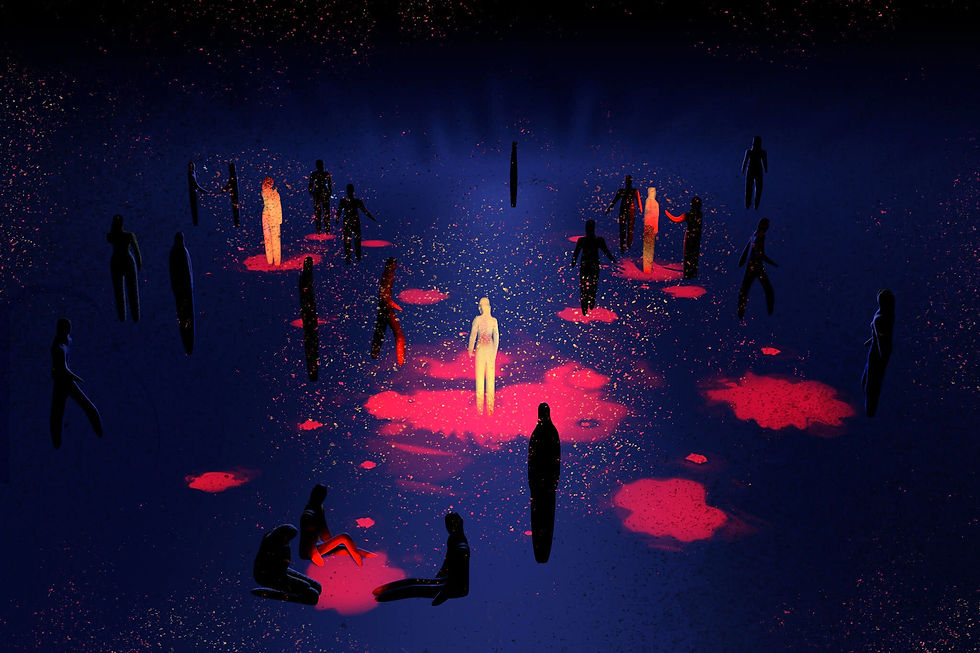
Portal of Entry: Host-Pathogen Interactions
The site at which an infectious agent enters a vulnerable host and makes contact with its tissues is called the portal of entry. Examples of entry points are the gastrointestinal, urinary and pulmonary systems as well as affected skin and mucous membrane surfaces, which are often equated with the exit ports (14.1A: Portals of Microbe Entry, 2022). For example, the influenza virus enters the new host's respiratory tract after exiting the original host's airway. In contrast, many pathogens that cause gastroenteritis take the so-called fecal-oral route, leaving the original host via feces, traveling through improperly cleaned hands to a vehicle such as food, drink, or utensils, to then enter a new host via its mouth (Church, 1986; de Graaf et al., 2017). In addition, some infectious agents can naturally enter a susceptible host through more than one portal. For example, the three forms of human anthrax can be distinguished according to the route of entry of the pathogen: cutaneous anthrax enters through the skin, while gastrointestinal and inhalation anthrax enter the host through ingestion or inhalation of spores (Simonsen & Chatterjee, 2023).
A vulnerable host, i.e., an individual who has been exposed to an infectious agent and is at risk of infection or developing disease serves as the last link in the infection chain. The host's genetic makeup is one of several elements that affect the host's susceptibility to infection (Understanding the Chain of Infection, 2023). This can, however, affect susceptibility in a beneficial or detrimental way. For example, people with sickle cell anemia, an inherited disease characterized by the production of sickle- or crescent-shaped red blood cells, are protected from severe malaria (Luzzatto, 2012). Other factors include specific or non-specific immunity which ultimately reflect an individual's ability to fight infection, as well as malnutrition, alcoholism or therapy that impairs the immune response. Anatomic and physiological barriers such as the skin, mucous membranes, respiratory cilia, stomach acidity and the cough reflex, are examples of nonspecific defense mechanisms (Humphrey & Perdue, 2023). On the other hand, antibodies that are protective and targeted at a particular pathogen lay the foundation for specific immunity. These antibodies may be produced in response to an infection, vaccination, or a toxoid (a toxin that has been rendered inactive but still has the ability to trigger the formation of antibodies), or they may be acquired by transfer from the mother to the fetus (Renneberg et al., 2017).

Breaking The Chain of Infection: Implications for Public Health
Understanding the exit and entry gates for infectious agents and their transmission networks lays the foundation for devising effective control measures. The best course of action for some disorders may involve reducing or removing the agent at its source. For instance, antibiotics may be used to treat a patient who has a bacterial infection in order to eradicate it. Asymptomatic yet infected people can be treated to clear the infection and limit the chance of infection transmission. Additionally, to prevent the agent from escaping from its reservoir, the soil can be decontaminated or coated (Principles of Epidemiology | Lesson 1 - Section 10, 2012). Some other approaches focus on the transmission route. Direct transmission between humans can be interrupted by isolating infected individuals (quarantine), by advising people to avoid some form of contact associated with disease transmission and by locating and monitoring healthy people who had come into contact with infected patients (Pandey et al., 2014). Measures to prevent fecal-oral transmission include hand washing, provision of clean water and adequate sanitation facilities, and promotion of personal and domestic hygiene to limit risks of contamination (Black et al., 2003; Prüss-Üstün et al., 2008). Airborne transmission can be controlled by adjusting ventilation, air pressure, and air filtration. One such example is negative pressure isolation rooms in hospitals, where exhaust vents can be used to manipulate airflow (Dowdall et al., 2010).
Vector-borne transmission, on the other hand, can be prevented by spraying the vector population with insecticides such as dichloro-diphenyl-trichloroethane (DDT), which was widely used to kill malaria-carrying mosquitos but has serious environmental consequences being banned in several countries (Kayawe, 2022). To specifically address the exit (and entry) portals of infectious agents in human reservoirs, infection control practices include hand hygiene and the use of personal protective equipment (PPE), which includes gloves, gowns and face masks (Siegel et al., 2007). Important prevention and control measures targeting the susceptible host include both prophylactic treatment, which can help stop infection from taking hold, and vaccines, which promote the production of specific antibodies against infection hence protecting the host. Importantly, individuals lacking immunity are indirectly protected when a significant proportion of the population develops immunity to an infectious disease, either through natural infection or through vaccination, as the rate of disease transmission becomes relatively low (Phadke et al., 2016). This phenomenon is known as herd immunity. Although paramount in the epidemiological context, the level of herd immunity required to prevent or stop an outbreak varies by disease, such that immunisation rates as high as 85% to 90% did not prevent measles and rubella outbreaks (Principles of Epidemiology | Lesson 1 - Section 10, 2012).

Conclusions
"It is time to close the book on infectious diseases and declare the pestilence war won", says Spellberg (2008). The COVID-19 pandemic was just a reminder that such words do not apply to the modern world. Public health professionals wage a never-ending battle against new infections, resurgences of old infections and the antibiotic crisis, as more and more bacteria become resistant to available antibiotics. With this in mind, it is crucial to develop the necessary tools to uncover the chain of infection and better understand transmission dynamics so that effective prevention and control strategies can be developed. The cornerstone of successful public health initiatives is laid by precise terminologies and a better understanding of the rationale underlying the emergence and establishment of each infection. This underscores the importance of understanding the chain of infection for each disease in order to provide the necessary tools for effective public health responses aimed at breaking each link in this chain.
Bibliographical References
Black, R. E., Morris, S. S., & Bryce, J. (2003). Where and why are 10 million children dying every year? The Lancet, 361(9376), 2226–2234. https://doi.org/10.1016/S0140-6736(03)13779-8
Burke, R. L., Kronmann, K. C., Daniels, C. C., Meyers, M., Byarugaba, D. K., Dueger, E., Klein, T. A., Evans, B. P., & Vest, K. G. (2012). A Review of Zoonotic Disease Surveillance Supported by the Armed Forces Health Surveillance Center. Zoonoses and Public Health, 59(3), 164–175. https://doi.org/10.1111/j.1863-2378.2011.01440.x
Church, J. (1986). Spread of infection: faecal oral route. Nursing, 3(4), 140–141. https://pubmed.ncbi.nlm.nih.gov/3635776/
de Graaf, M., Beck, R., Caccio, S. M., Duim, B., Fraaij, P. LA, Le Guyader, F. S., Lecuit, M., Le Pendu, J., de Wit, E., & Schultsz, C. (2017). Sustained fecal-oral human-to-human transmission following a zoonotic event. Current Opinion in Virology, 22, 1–6. https://doi.org/10.1016/j.coviro.2016.11.001
Delamater, P. L., Street, E. J., Leslie, T. F., Yang, Y. T., & Jacobsen, K. H. (2019). Complexity of the Basic Reproduction Number (R0). Emerging Infectious Diseases, 25(1), 1–4. https://doi.org/10.3201/eid2501.171901
Dowdall, N. P., Evans, A. D., & Thibeault, C. (2010). Air Travel and TB: An airline perspective. Travel Medicine and Infectious Disease, 8(2), 96–103. https://doi.org/10.1016/j.tmaid.2010.02.006
Farnsworth, C. (2022). What are bacterial meningitis droplet precautions? Medical News Today.
Gunderson, A., & Woskie, L. (2020). Understanding Predictions: What is R-Naught? Harvard Global Health Institute. https://bio.libretexts.org/Bookshelves/Microbiology/Microbiology_(Boundless)/14%3A_Pathogenicity/14.01%3A_Entry_into_the_Host/14.1A%3A_Portals_of_Microbe_Entry
Hinnebusch, B. J., & Erickson, D. L. (2008). Yersinia pestis Biofilm in the Flea Vector and Its Role in the Transmission of Plague (pp. 229–248). https://doi.org/10.1007/978-3-540-75418-3_11
Humphrey, J. H., & Perdue, S. S. (2023). immune system. In Encyclopedia Britannica.
In the News. (2009). Nature Reviews Microbiology, 7(9), 622–622. https://doi.org/10.1038/nrmicro2215
Jr., S. C. O., Mitchell, V. S., Pearson, G. W., & Carpenter, C. C. J. (1991). Malaria: Obstacles and Opportunities. National Academies Press. https://doi.org/10.17226/1812
Kayawe, B. (2022). Widespread use of DDT for malaria control worries environmentalist. Africa Renewal. https://www.un.org/africarenewal/magazine/january-2022/widespread-use-ddt-malaria-control-worries-environmentalist
Leavitt, J. W. (1996). Typhoid Mary: captive to the public’s health. Beacon Press.
Louten, J. (2016). Virus Transmission and Epidemiology. In Essential Human Virology (pp. 71–92). Elsevier. https://doi.org/10.1016/B978-0-12-800947-5.00005-3
Luzzatto, L. (2012). Sickle cell anemia and malaria. Mediterranean Journal of Hematology and Infectious Diseases, 4(1), e2012065. https://doi.org/10.4084/mjhid.2012.065
Marineli, F., Tsoucalas, G., Karamanou, M., & Androutsos, G. (2013). Mary Mallon (1869-1938) and the history of typhoid fever. Annals of Gastroenterology, 26(2), 132–134. http://www.ncbi.nlm.nih.gov/pubmed/24714738
Pandey, A., Atkins, K. E., Medlock, J., Wenzel, N., Townsend, J. P., Childs, J. E., Nyenswah, T. G., Ndeffo-Mbah, M. L., & Galvani, A. P. (2014). Strategies for containing Ebola in West Africa. Science, 346(6212), 991–995. https://doi.org/10.1126/science.1260612
Peck, M. W. (2014). Bacteria: Clostridium botulinum. In Encyclopedia of Food Safety (pp. 381–394). Elsevier. https://doi.org/10.1016/B978-0-12-378612-8.00091-3
Phadke, V. K., Bednarczyk, R. A., Salmon, D. A., & Omer, S. B. (2016). Association Between Vaccine Refusal and Vaccine-Preventable Diseases in the United States. JAMA, 315(11), 1149. https://doi.org/10.1001/jama.2016.1353
Portals of Microbe Entry. (2022). LibreTexts Biology. https://bio.libretexts.org/Bookshelves/Microbiology/Microbiology_(Boundless)/14%3A_Pathogenicity/14.01%3A_Entry_into_the_Host/14.1A%3A_Portals_of_Microbe_Entry
Principles of Epidemiology | Lesson 1 - Section 10. (2012). CDC. https://www.cdc.gov/csels/dsepd/ss1978/lesson1/section10.html#:~:text=More%20specifically%2C%20transmission%20occurs%20when,called%20the%20chain%20of%20infection.
Prüss-Üstün, A., Bos, R., Gore, F., & Bartram, J. (2008). Safer water, better health. World Health Organization, 53. http://www.who.int/quantifying_ehimpacts/publications/saferwater/en/
Ramirez, V. B. (2023). What Is “R-naught”? Gauging Contagious Infections. Healthline. https://www.healthline.com/health/r-naught-reproduction-number
Renneberg, R., Berkling, V., & Loroch, V. (2017). Viruses, Antibodies, and Vaccines. In Biotechnology for Beginners (pp. 165–200). Elsevier. https://doi.org/10.1016/B978-0-12-801224-6.00005-9
Ryu, S., Chun, J. Y., Lee, S., Yoo, D., Kim, Y., Ali, S. T., & Chun, B. C. (2022). Epidemiology and Transmission Dynamics of Infectious Diseases and Control Measures. Viruses, 14(11), 2510. https://doi.org/10.3390/v14112510
Sattar, S. A., Tetro, J., Bidawid, S., & Farber, J. (2000). Foodborne Pread of Hepatitis A: Recent Studies on Virus Survival, Transfer and Inactivation. Canadian Journal of Infectious Diseases, 11(3), 159–163. https://doi.org/10.1155/2000/805156
Shaklee, J. (2012). Pertussis Precautions for Professionals. Children’s Hospital of Philadelphia. https://www.chop.edu/pages/pertussis-precautions-professionals
Siegel, J. D., Rhinehart, E., Jackson, M., & Chiarello, L. (2007). 2007 Guideline for Isolation Precautions: Preventing Transmission of Infectious Agents in Health Care Settings. American Journal of Infection Control, 35(10), S65–S164. https://doi.org/10.1016/j.ajic.2007.10.007
Simonsen, K. A., & Chatterjee, K. (2023). Anthrax. Treasure Island (FL): StatPearls Publishing.
Spellberg, B. (2008). Dr. William H. Stewart: Mistaken or Maligned? Clinical Infectious Diseases, 47(2), 294–294. https://doi.org/10.1086/589579
Strochlic, N. (2020). Typhoid Mary’s tragic tale exposed the health impacts of “super-spreaders.” National Geographic. https://www.nationalgeographic.com/history/article/typhoid-mary-tragic-tale-exposed-health-impacts-super-spreaders
Tulchinsky, T. H., & Varavikova, E. A. (2014). Communicable Diseases. In The New Public Health (pp. 149–236). Elsevier. https://doi.org/10.1016/B978-0-12-415766-8.00004-5
Understanding the Chain of Infection. (2023). ATrain Education. https://www.atrainceu.com/content/2-understanding-chain-infection
van Seventer, J. M., & Hochberg, N. S. (2017). Principles of Infectious Diseases: Transmission, Diagnosis, Prevention, and Control. In International Encyclopedia of Public Health (pp. 22–39). Elsevier. https://doi.org/10.1016/B978-0-12-803678-5.00516-6
Wang, C. C., Prather, K. A., Sznitman, J., Jimenez, J. L., Lakdawala, S. S., Tufekci, Z., & Marr, L. C. (2021). Airborne transmission of respiratory viruses. Science, 373(6558). https://doi.org/10.1126/science.abd9149
Yadav, A., Kumar, S., Singh, G., & Kansara, N. (2021). Demystifying R naught: Understanding what does it hide? Indian Journal of Community Medicine, 46(1), 7. https://doi.org/10.4103/ijcm.IJCM_989_20
Yu, I. T. S., Li, Y., Wong, T. W., Tam, W., Chan, A. T., Lee, J. H. W., Leung, D. Y. C., & Ho, T. (2004). Evidence of Airborne Transmission of the Severe Acute Respiratory Syndrome Virus. New England Journal of Medicine, 350(17), 1731–1739. https://doi.org/10.1056/NEJMoa032867
Visual Sources
Figure 1: Strochlic, N. (2020). Typhoid Mary’s tragic tale exposed the health impacts of “super-spreaders.” [Image]. National Geographic. https://www.nationalgeographic.com/history/article/typhoid-mary-tragic-tale-exposed-health-impacts-super-spreaders
Figure 2: Jones, B. (2021). Where could the next coronavirus jump to humans? New research offers clues. [Image]. Vox. https://www.vox.com/22456392/coronavirus-pandemic-bats-forests-spillover-china-indonesia
Figure 3: Jarvis, B. (2019). How Mosquitoes Changed Everything. [Image]. The New Yorker. https://www.newyorker.com/magazine/2019/08/05/how-mosquitoes-changed-everything
Figure 4: Moyer, M. W. (2021). What are the symptoms of Omicron? [Image]. The New York Times. https://www.nytimes.com/2021/12/21/well/live/omicron-variant-symptoms-covid.html
Figure 5: Weerasekera, N. (2023). Not Every Pandemic Needs Someone to Blame. [Image]. The New York Times. https://www.nytimes.com/2023/05/21/opinion/the-next-pandemic-blame.html
Figure 6: Huertas, K. (2022). 2022 was the year long COVID couldn’t be ignored. [Image]. Science News. https://www.sciencenews.org/article/2022-long-covid-lingering-symptoms-treatments
Comments