Familial Hypercholesterolaemia: Genetics of Cardiovascular Disease
Cholesterol, a lipid which exists in both an endogenous and exogenous form, garners a negative reputation due to its link with cardiovascular disease (CVD). Although a high fat diet is considered the prime culprit in increasing cholesterol levels (Carson et al., 2020), around 70% of the body’s cholesterol is actually accounted for by de novo synthesis in the liver (Kapourchali et al., 2015). Cholesterol transport is a multifaceted, physiological process which involves 2 main pathways. The exogenous lipid pathway begins in the gastrointestinal tract and involves the transport of lipids and fatty acids, derived from our diet, to the liver in tightly packed particles called chylomicrons. Then, an endogenous lipid pathway transforms remnants of chylomicrons in the liver into particles that have lower densities; this is achieved by removing contents like triglycerides and surface molecules called apoliproteins (Feingold and Grunfield, 2018). Very low density lipoproteins (VLDL), intermediate density lipoproteins (IDL) and low density lipoproteins (LDL) are formed in this pathway. The lipid-carrying particle which is most commonly associated with CVD is LDL (Yoon et al., 2021). LDL predominantly consists of free cholesterol and has the ability to deliver it to peripheral tissues, hence promoting the build-up of cholesterol deposits in tissues (Rae et al., 2018).
Homeostasis in the body is regulated by balancing the synthesis and excretion of cholesterol through the individual transport systems and the enzymatic processes associated with them (Yoon et al., 2021). A disruption in homeostasis can lead to conditions which cause abnormally high levels of lipoproteins and cholesterol in the body (Duan et al., 2022). Familial hypercholesterolaemia (FH) is one such condition. FH is an inherited dyslipidemia which manifests as elevated levels of LDL-cholesterol. Elevation of LDL increases the risk of cholesterol deposition in blood vessel walls. Cholesterol deposits in arteries can result in atherosclerosis, the formation of plaques and narrowing of arteries which leads to myocardial infarctions (MI) and coronary artery disease (CAD) (Yu et al., 2022). Due to this, FH dramatically elevates the risk that an affected indivual will suffer from a CVD event. According to a meta-analyses involving 11 million patients, it is suspected that 1 in 313 people suffer from the condition, although only 30% of them receive a formal diagnosis (Beheshti et al., 2020). FH is an autosomal dominant genetic disorder, meaning one mutated allele is sufficient to cause the disease; this form of inheritance also means that there is a 50% chance that the offspring of an affected individual will have FH (Vrablik et al., 2020).
This article will describe the pathophysiology of FH and outline signs that can help a person recognise if them or their loved ones are affected by this condition. It will also list diagnosis criteria that can be used to identify FH and evaluate FH treatments that help avoid fatal CVD events.
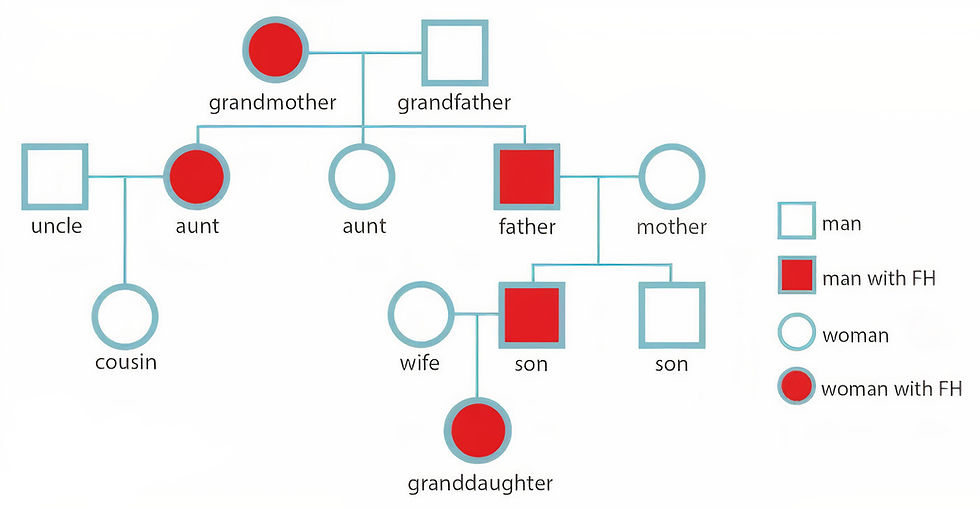
Figure 1: Pedigree showing the inheritance of FH (Family Heart Foundation, n.d)
Genetics of FH
FH can present itself as a heterozygous or homozygous disorder, with the latter being a more severe but rarer genotype. Classical manifestation of FH is described to be monogenic, caused by a single mutation in either the LDLR, PCSK9 or the APOB gene (Sharifi et al., 2017). The Centers for Disease Control and Prevention (2022) estimates that between 60% and 80% of individuals diagnosed with FH have an identifiable defect in one of the listed genes. The LDLR gene encodes for the LDL receptor. LDLR is expressed on the surface of cells to regulate the uptake of LDL particles in the endogenous lipid pathway. This maintains LDL levels in the blood and allows for cholesterol to be utilised for cellular activity instead of depositing in tissues (Feingold & Grunfeld, 2018). A mutation in LDLR is the most common defect which can cause FH, with mutations being caused by deletions or duplication of sections of the gene as well as single base substitutions which alter amino acids encoded by the gene. An aberrant LDLR gene results in a non-functional LDL receptor. This in turn reduces the amount of LDL that can be taken up into cells, leaving more LDL-cholesterol circulating in the blood (Sharifi et al., 2017).
PCSK9 (proprotein convertase subtilisin/Kexin type 9) is an enzyme predominantly found in hepatocytes which disrupts the recycling of LDLR. PCSK9 marks surface LDLR for degradation by lysosomes inside the cells to reduce the number of available LDLRs that can be re-expressed on the cell surface (Feingold & Grunfeld, 2018). In some FH patients, this physiological pathway is enhanced by a gain-of-function PCSK9 mutation. An increase in PSCK9 results in the destruction of more LDLRs than normal and consequently, dramatically reduces the uptake of LDL molecules into the liver (Di Taranto & Fortunato, 2023).. Therefore, PCSK9 is another gene implicated in causing the hypercholesterolaemia observed in FH.
Finally, APOB mutations have also been identified as monogenic causes of FH. The APOB gene is responsible for the production of apoliproteinB100 (ApoB100), a ligand of LDLR expressed on the surface of LDL molecules. ApoB100 interaction with LDLR is required for the internalisation of LDL into the liver (Feingold & Grunfeld, 2018). In FH, the ApoB100 protein can be defective and hence, prevent intake of LDL into body cells and the liver. This paves way for the accumulation of LDL-cholesterol in the blood and peripheral tissues (Abifadel & Boileau, 2022).
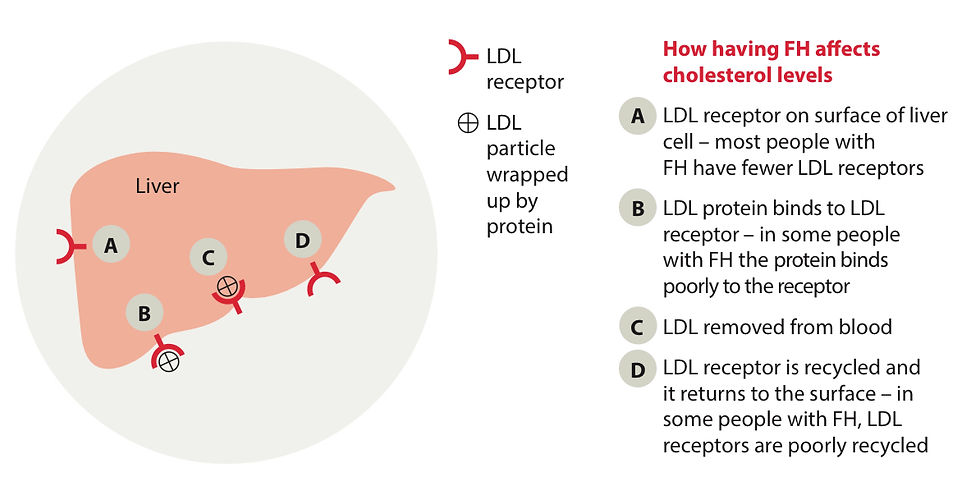
Figure 2: A diagram summarising the pathophysiology of monogenic FH (British Heart Foundation, n.d)
However, it is known that individuals without a mutation in the 3 genes mentioned can still inherit FH. Hence, it is suspected that FH can also be polygenic, meaning that inputs from different mutated genes all summate to cause the raised LDL and cholesterol levels seen in affected patients (Talmud et al., 2013). Across multiple genetic screenings of affected individuals, APOE, SORT1 and ABCG8 genes have been identified as potential sites of mutations which cause polygenic FH (Feingold & Grunfeld, 2018; Mohd Nor et al., 2019). DNA methylation, a post-translational modification which can change gene function, has also been studied as an underlying mechanism for polygenic FH. Researcher Reeskamp and colleagues analysed the DNA methylation of genes involved in lipid processing in patients with mutation-negative FH. It was found that these patients had differentially methylated sites across the genome when compared to patients with monogenic FH, with significant hypomethylation identified in the CPT1A gene (Reeskamp et al., 2020). Yet, further research is required to elucidate the complete pathophysiology of FH which is not caused by a mutation in either LDLR, PCSK9 or APOB gene.
Diagnosing FH early to minimise fatal consequences
A rise in LDL cholesterol is correlated with a higher CVD risk. Studies have continuously shown that elevations in LDL cholesterol result in higher incidence of MI, CAD and CVD, even in individuals who are labelled to be at a low risk of dying from CVD (Abdullah et al., 2018). Hence, due to the marked elevation in LDL, suffering a cardiovascular event can be up to 20x more likely in patients with FH compared to the unaffected population (Krogh et al., 2016).
Due to the fatal outcomes, it is crucial to detect FH and begin treatment as soon as possible. There are currently 3 widely used diagnostic criteria for FH: The Simon-Broom criteria, the Dutch criteria and the US MEDPED criteria (Heart UK, n.d). Diagnosis typically takes into account an individual’s lipid profile - placing an emphasis on total cholesterol and LDL-cholesterol levels - family history, symptomatology and genotype. Premature (before 55 years in men and before 60 years in women) CVD in either patient or family member often constitutes as evidence of adverse family history (Al-Rasadi et al., 2014). Physical manifestations such as tendon xanthomas, corneal arcus and xanthelasmas found near the eye can be giveaway symptoms of FH which a physician can detect (Vaezi & Amini, 2020).
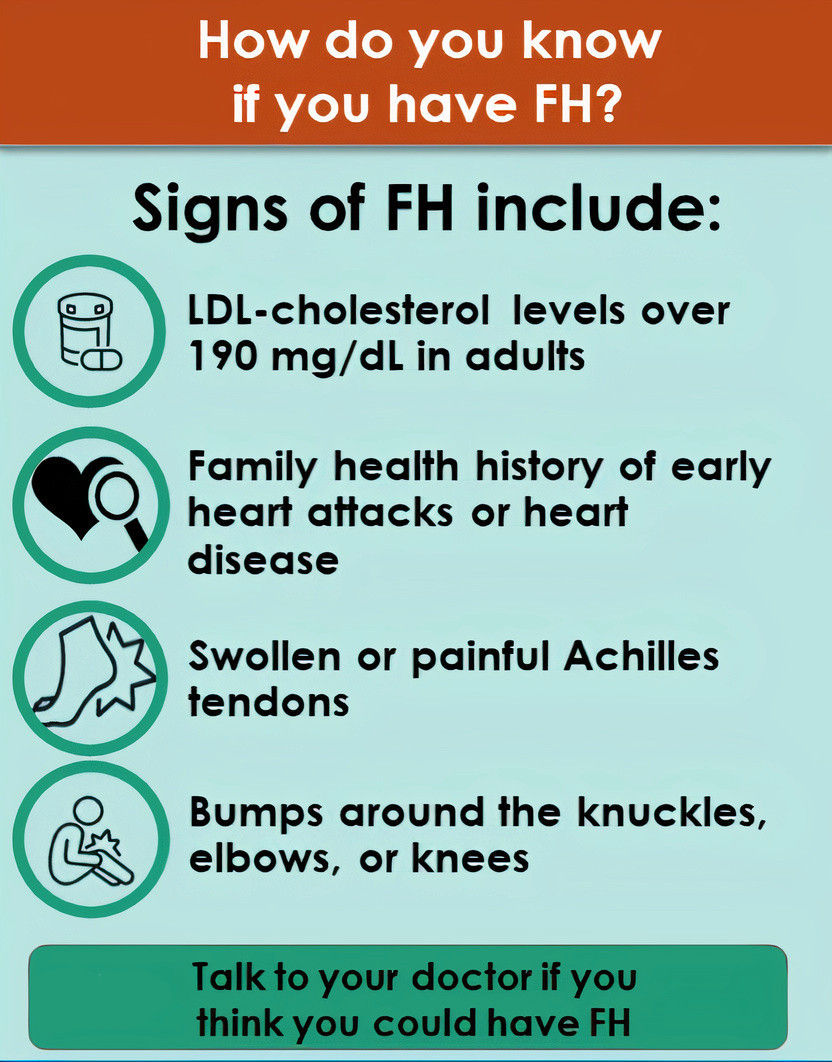
Figure 3: Infographic describing the signs of FH (Centers for Disease Control and Prevention, 2022)
Different screening models have been implemented globally for identifying people with FH. Lipid screening is done to identify individuals with LDL cholesterol and total cholesterol levels which exceed the standardised range. Evaluating the lipid profile is common in paediatric patients as it allows FH to be identified early on in life (Scicali et al., 2018). Cascade screening is another model for identifying FH. It uses confirmatory genetic testing to identify an index patient with FH who has a mutation in one of the 3 genes previously discussed. Then, screening of first, second and third degree relatives of the index patient proceeds to diagnose FH in as many family members as possible (Besseling et al., 2015). Genetic testing is now being viewed as a more desirable option in identifying FH due to the rise of new generation sequencing which has the power to detect novel mutations. Government schemes should therefore push to implement genetic screening in their country’s health plan so that more individuals with FH can be identified (Rutkowska et al., 2022).
Current and future treatments of FH
FH cannot be cured by eliminating a high fat diet and leading an active life style, although both changes are recommended to be made in conjunction with treatment (Public Health England et al., 2018). The current first-line treatment of FH is statin treatment. Statins are drugs which target the synthesis pathway of cholesterol. They specifically inhibit hydroxymethylglutayl-CoA (HMG-CoA) reductase enzyme, reducing endogenous cholesterol production. Thus, statins are a form of cholesterol lowering drug (Sirtori, 2014). An evaluation of 22 clinical trials has shown that CVD occurrence in adults was reduced by statin therapy when compared to controls taking a placebo drug (Chou et al., 2022). Furthermore, children diagnosed with FH who were started on a statin regime and followed up for 20 years had shown a 32% reduction in LDL cholesterol since the initiation of treatment, had reduced progression of artery wall thickening and possessed a CVD risk lower than in their affected parents. Hence, statins are crucial for promoting longevity and health in FH patients (Luirink et al., 2019).

Figure 4: Graph displaying the age range at with CVD manifests in heterozygous and homozygous FH individuals, with and without cholesterol-lowering therapy (Bianconi et al., 2020)
PCSK9 inhibitors are a type of more recent pharmaceuticals used to treat severe FH. Monoclonal antibodies are proteins which are able to bind to PCSK9 enzymes with high-affinity and neutralise them (Tomlinson et al., 2021). Small interfering RNA can also inhibit PCSK9 by blocking its production at the stage of mRNA translation (Wołowiec et al., 2023). Thus, this class of drugs prevents PCSK9-dependent degradation of LDLR. This causes a rise in LDLR expression, positively impacting patients with FH as more LDL can be internalised and metabolised by cells. Although clinical trials have shown up to 60% reductions in LDL cholesterol after 12 weeks of consistent PCSK9 inhibitor use (Jia et al., 2022), health guidelines recommend PCSK9 use only in circumstances when a patient is at an extremely high risk of CVD (Hao et al., 2022).
For patients with homozygous FH and heterozygous FH which is refractory to first-line therapies, drug treatments are rarely potent enough to produce a significant cholesterol-lowering effect. Therefore, lipoprotein apheresis becomes a therapeutic option (Family Heart Foundation, n.d). The procedure involves a filtration process which passes a patient’s blood through a machine that selectively removes lipoproteins from it before circulating the blood back into the patient (Makino et al., 2019). After monitoring 14 patients with FH who had undertaken regular lipoprotein apheresis for 15 years, Víšek and colleagues showed that the procedure effectively reduced LDL and total cholesterol while also playing a role in removing inflammatory markers which aggravated damage to blood vessel walls (Jan Ámos Víšek et al., 2021). Overall, lipoprotein apheresis is suggested to lead to a better prognosis in patients with FH, though research emphasises that benefits are most evident when treatment is commenced during childhood (Græsdal et al., 2012).
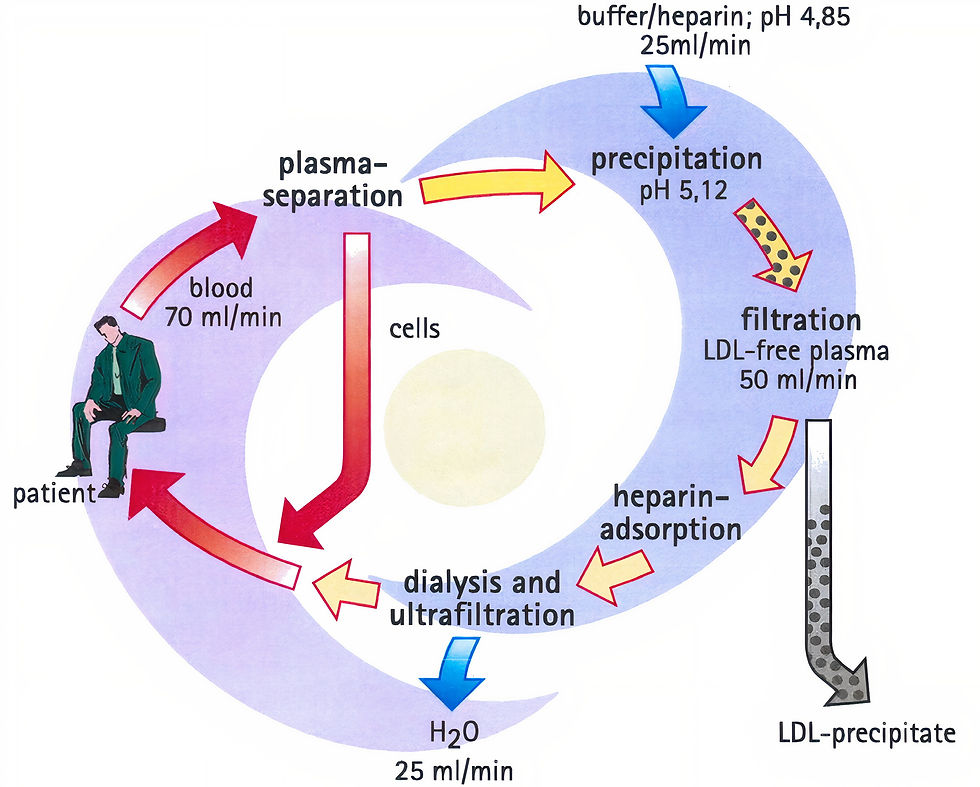
Figure 5: A diagram summarising the process of lipoprotein apheresis (McGowan, 2013)
The search for FH therapeutics is still ongoing as current treatments are limited by side-effects, high cost and the inability to be effective in all populations (Lui et al., 2020). A team of researchers attempted to test the cholesterol-lowering abilities of a traditional Pu-erh tea. Mice on a high-fat diet were used as models of hypercholesterolaemia. The results showed that after a period of 26 weeks of having Pu-erh tea in their diet, the mice were observed to have a reduction in blood lipids due to an altered gut microbiota and bile excretion pathway. Future efforts can be used to explore the effects of the Pu-erh tea in humans while also investigating the role of the gut microbiota in cholesterol homeostasis (Huang et al., 2019). Furthermore, Verve Therapeutics have initiated a phase 1 trial of genetic-editing technology which intends to silence the expression of the PCSK9 gene in order to diminish the PCSK9 molecules available to degrade LDLRs. Although genetic manipulations are a more invasive method of treatment, they offer the potential to cure FH if the mutated gene is recovered or silenced (Verve Therapeutics, Inc., 2023).
Conclusions
To conclude, FH is a genetic condition which causes a stark elevation in LDL cholesterol and heightens an individual’s risk of experiencing a CVD event, which can prove fatal. Although prevalent, FH is still substantially under-diagnosed worldwide. This article was written to raise awareness on the existence of the condition, encourage individuals to be tested and inform those affected of what therapeutics are available which can prolong and improve their lives. Efforts need to be made so that genetic screening for FH becomes a feasible option worldwide. Additionally, current treatments, as well as those that are being developed, need to be affordable and accessible to everyone to promote health equity and equality. Finally, more outreach needs to be done to ensure that FH becomes a condition that is acknowledged and receives the attention which is necessary to effectively manage it.
Bibliographical References
Abdullah, S. M., Defina, L. F., Leonard, D., Barlow, C. E., Radford, N. B., Willis, B. L., Rohatgi, A., McGuire, D. K., de Lemos, J. A., Grundy, S. M., Berry, J. D., & Khera, A. (2018). Long-Term Association of Low-Density Lipoprotein Cholesterol With Cardiovascular Mortality in Individuals at Low 10-Year Risk of Atherosclerotic Cardiovascular Disease. Circulation, 138(21), 2315–2325. https://doi.org/10.1161/circulationaha.118.034273
Abifadel, M., & Boileau, C. (2022). Genetic and molecular architecture of familial hypercholesterolemia. Journal of Internal Medicine, 293(2), 144–165. https://doi.org/10.1111/joim.13577
Al-Rasadi, K., Al-Waili, K., Al-Sabti, H. A., Al-Hinai, A., Al-Hashmi, K., Al-Zakwani, I., & Banerjee, Y. (2014). Criteria for Diagnosis of Familial Hypercholesterolemia: A Comprehensive Analysis of the Different Guidelines, Appraising their Suitability in the Omani Arab Population. Oman Medical Journal, 29(2), 85–91. https://doi.org/10.5001/omj.2014.22
Beheshti, S. O., Madsen, C. M., Varbo, A., & Nordestgaard, B. G. (2020). Worldwide Prevalence of Familial Hypercholesterolemia: Meta-Analyses of 11 Million Subjects. Journal of the American College of Cardiology, 75(20), 2553–2566. https://doi.org/10.1016/j.jacc.2020.03.057
Besseling, J., Sjouke, B., & Kastelein, J. J. P. (2015). Screening and treatment of familial hypercholesterolemia – Lessons from the past and opportunities for the future (based on the Anitschkow Lecture 2014). Atherosclerosis, 241(2), 597–606. https://doi.org/10.1016/j.atherosclerosis.2015.06.011
Carson, J. A. S., Lichtenstein, A. H., Anderson, C. A. M., Appel, L. J., Kris-Etherton, P. M., Meyer, K. A., Petersen, K., Polonsky, T., & Van Horn, L. (2020). Dietary Cholesterol and Cardiovascular Risk: A Science Advisory From the American Heart Association. Circulation, 141(3). https://doi.org/10.1161/cir.0000000000000743
Centers for Disease Control and Prevention. (2022). Heart Disease, Family Health History, and Familial Hypercholesterolemia. https://www.cdc.gov/genomics/disease/fh/FH.htm
Chou, R., Cantor, A., Dana, T., Wagner, J., Ahmed, A. Y., Fu, R., & Ferencik, M. (2022). Statin Use for the Primary Prevention of Cardiovascular Disease in Adults. JAMA, 328(8), 754. https://doi.org/10.1001/jama.2022.12138
Di Taranto, M. D., & Fortunato, G. (2023). Genetic Heterogeneity of Familial Hypercholesterolemia: Repercussions for Molecular Diagnosis. International Journal of Molecular Sciences, 24(4), 3224. https://doi.org/10.3390/ijms24043224
Duan, Y., Gong, K., Xu, S., Zhang, F., Meng, X., & Han, J. (2022). Regulation of cholesterol homeostasis in health and diseases: from mechanisms to targeted therapeutics. Signal Transduction and Targeted Therapy, 7(1), 1–29. https://doi.org/10.1038/s41392-022-01125-5
Family Heart Foundation. (n.d). Lipoprotein Apheresis. https://familyheart.org/fh-treatments/lipoprotein-apheresis
Feingold, K. R., & Grunfeld, C. (2018, February 2). Introduction to Lipids and Lipoproteins. Nih.gov; MDText.com, Inc. https://www.ncbi.nlm.nih.gov/books/NBK305896/
Græsdal, A., Bogsrud, M. P., Holven, K. B., Nenseter, M. S., Narverud, I., Langslet, G., Brekke, M., Retterstøl, K., Arnesen, K.-E., & Ose, L. (2012). Apheresis in homozygous familial hypercholesterolemia: The results of a follow-up of all Norwegian patients with homozygous familial hypercholesterolemia. Journal of Clinical Lipidology, 6(4), 331–339. https://doi.org/10.1016/j.jacl.2012.03.004
Hao, Q., Aertgeerts, B., Guyatt, G., Bekkering, G. E., Vandvik, P. O., Khan, S. U., Rodondi, N., Jackson, R., Reny, J.-L., Al Ansary, L., Van Driel, M., Assendelft, W. J. J., Agoritsas, T., Spencer, F., Siemieniuk, R. A. C., Lytvyn, L., Heen, A. F., Zhao, Q., Riaz, I. B., & Ramaekers, D. (2022). PCSK9 inhibitors and ezetimibe for the reduction of cardiovascular events: a clinical practice guideline with risk-stratified recommendations. BMJ (Clinical Research Ed.), 377, e069066. https://doi.org/10.1136/bmj-2021-069066
Heart UK The Cholesterol Charity. (n.d). Diagnosing FH. https://www.heartuk.org.uk/fh/diagnosing-fh
Huang, F., Zheng, X., Ma, X., Jiang, R., Zhou, W., Zhou, S., Zhang, Y., Lei, S., Wang, S., Kuang, J., Han, X., Wei, M., You, Y., Li, M., Li, Y., Liang, D., Liu, J., Chen, T., Yan, C., & Wei, R. (2019). Theabrownin from Pu-erh tea attenuates hypercholesterolemia via modulation of gut microbiota and bile acid metabolism. Nature Communications, 10(1), 4971. https://doi.org/10.1038/s41467-019-12896-x
Jan Ámos Víšek, Bláha, M., Vladimír Bláha, M. Lasticova, Lánská, M., Ctirad Andrýs, Jurjen Duintjer Tebbens, Igreja, C., K. Tripská, M. Vicen, Iveta Najmanová, & Nachtigal, P. (2021). Monitoring of up to 15 years effects of lipoprotein apheresis on lipids, biomarkers of inflammation, and soluble endoglin in familial hypercholesterolemia patients. 16(1). https://doi.org/10.1186/s13023-021-01749-w
Jia, X., Al Rifai, M., Saeed, A., Ballantyne, C. M., & Virani, S. S. (2022). PCSK9 Inhibitors in the Management of Cardiovascular Risk: A Practical Guidance. Vascular Health and Risk Management, Volume 18, 555–566. https://doi.org/10.2147/vhrm.s275739
Kapourchali, F. R., Surendiran, G., Goulet, A., & Moghadasian, M. H. (2015). The Role of Dietary Cholesterol in Lipoprotein Metabolism and Related Metabolic Abnormalities: A Mini-review. Critical Reviews in Food Science and Nutrition, 56(14), 2408–2415. https://doi.org/10.1080/10408398.2013.842887
Krogh, H. W., Mundal, L., Holven, K. B., & Retterstøl, K. (2016). Patients with familial hypercholesterolaemia are characterized by presence of cardiovascular disease at the time of death. European Heart Journal, 37(17), 1398–1405. https://doi.org/10.1093/eurheartj/ehv602
Lui, D. T. W., Lee, A. C. H., & Tan, K. C. B. (2020). Management of Familial Hypercholesterolemia: Current Status and Future Perspectives. Journal of the Endocrine Society, 5(1), bvaa122. https://doi.org/10.1210/jendso/bvaa122
Luirink, I. K., Wiegman, A., Kusters, D. M., Hof, M. H., Groothoff, J. W., de Groot, E., Kastelein, J. J. P., & Hutten, B. A. (2019). 20-Year Follow-up of Statins in Children with Familial Hypercholesterolemia. New England Journal of Medicine, 381(16), 1547–1556. https://doi.org/10.1056/nejmoa1816454
Makino, H., Koezuka, R., Tamanaha, T., Ogura, M., Matsuki, K., Hosoda, K., & Harada-Shiba, M. (2019). Familial Hypercholesterolemia and Lipoprotein Apheresis. Journal of Atherosclerosis and Thrombosis, 26(8), 679–687. https://doi.org/10.5551/jat.RV17033
Mohd Nor, N. S., Al-Khateeb, A. M., Chua, Y.-A., Mohd Kasim, N. A., & Mohd Nawawi, H. (2019). Heterozygous familial hypercholesterolaemia in a pair of identical twins: a case report and updated review. BMC Pediatrics, 19(1). https://doi.org/10.1186/s12887-019-1474-y
Public Health England, National Institute for Health and Care Excellence , & National Health System England . (2018). Familial Hypercholesterolaemia Implementing a systems approach to detection and management [Review of Familial Hypercholesterolaemia Implementing a systems approach to detection and management ]. https://assets.publishing.service.gov.uk/government/uploads/system/uploads/attachment_data/file/731873/familial_hypercholesterolaemia_implementation_guide.pdf
Rae, P., Crane, M., & Pattenden, R. (2018). Clinical biochemistry. Wiley Blackwell.
Reeskamp, L. F., Venema, A., Pereira, J. P. B., Levin, E., Nieuwdorp, M., Groen, A. K., Defesche, J. C., Grefhorst, A., Henneman, P., & Hovingh, G. K. (2020). Differential DNA methylation in familial hypercholesterolemia. EBioMedicine, 61, 103079. https://doi.org/10.1016/j.ebiom.2020.103079
Rutkowska, L., Sałacińska, K., Salachna, D., Matusik, P., Pinkier, I., Kępczyński, Ł., Piotrowicz, M., Starostecka, E., Lewiński, A., & Gach, A. (2022). Identification of New Genetic Determinants in Pediatric Patients with Familial Hypercholesterolemia Using a Custom NGS Panel. Genes, 13(6), 999. https://doi.org/10.3390/genes13060999
Scicali, R., Di Pino, A., Platania, R., Purrazzo, G., Ferrara, V., Giannone, A., Urbano, F., Filippello, A., Rapisarda, V., Farruggia, E., Piro, S., Rabuazzo, A. M., & Purrello, F. (2018). Detecting familial hypercholesterolemia by serum lipid profile screening in a hospital setting: Clinical, genetic and atherosclerotic burden profile. Nutrition, Metabolism, and Cardiovascular Diseases: NMCD, 28(1), 35–43. https://doi.org/10.1016/j.numecd.2017.07.003
Sharifi, M., Futema, M., Nair, D., & Humphries, S. E. (2017). Genetic Architecture of Familial Hypercholesterolaemia. Current Cardiology Reports, 19(5). https://doi.org/10.1007/s11886-017-0848-8
Sirtori, C. R. (2014). The pharmacology of statins. Pharmacological Research, 88, 3–11. https://doi.org/10.1016/j.phrs.2014.03.002
Talmud, P. J., Shah, S., Whittall, R., Futema, M., Howard, P., Cooper, J. A., Harrison, S. C., Li, K., Drenos, F., Karpe, F., Neil, H. A. W., Descamps, O. S., Langenberg, C., Lench, N., Kivimaki, M., Whittaker, J., Hingorani, A. D., Kumari, M., & Humphries, S. E. (2013). Use of low-density lipoprotein cholesterol gene score to distinguish patients with polygenic and monogenic familial hypercholesterolaemia: a case-control study. The Lancet, 381(9874), 1293–1301. https://doi.org/10.1016/s0140-6736(12)62127-8
Tomlinson, B., Patil, N. G., Fok, M., & Lam, C. W. K. (2021). Role of PCSK9 Inhibitors in Patients with Familial Hypercholesterolemia. Endocrinology and Metabolism, 36(2), 279–295. https://doi.org/10.3803/enm.2021.964
Vaezi, Z., & Amini, A. (2020). Familial Hypercholesterolemia. PubMed; StatPearls Publishing. https://www.ncbi.nlm.nih.gov/books/NBK556009/
Verve Therapeutics, Inc. (2023, January 10). Open-label, Phase 1b, Single-ascending Dose and Optional re Dosing Study to Evaluate the Safety of VERVE-101 Administered to Patients With Heterozygous Familial Hypercholesterolemia, Atherosclerotic Cardiovascular Disease, and Uncontrolled Hypercholesterolemia. Clinicaltrials.gov. https://clinicaltrials.gov/ct2/show/NCT05398029?term=verve-101&draw=2&rank=1
Vrablik, M., Tichý, L., Freiberger, T., Blaha, V., Satny, M., & Hubacek, J. A. (2020). Genetics of Familial Hypercholesterolemia: New Insights. Frontiers in Genetics, 11. https://doi.org/10.3389/fgene.2020.574474
Wołowiec, Ł., Osiak, J., Wołowiec, A., Wijata, A., Grześk, E., Kozakiewicz, M., Banach, J., Nowaczyk, A., Nowaczyk, J., & Grześk, G. (2023). Inclisiran—Safety and Effectiveness of Small Interfering RNA in Inhibition of PCSK-9. Pharmaceutics, 15(2), 323. https://doi.org/10.3390/pharmaceutics15020323
Yoon, H., Shaw, J. L., Haigis, M. C., & Greka, A. (2021). Lipid metabolism in sickness and in health: Emerging regulators of lipotoxicity. Molecular Cell, 81(18), 3708–3730. https://doi.org/10.1016/j.molcel.2021.08.027
Yu, Y., Chen, L., Zhang, H., Fu, Z., Liu, Q., Zhao, H., Liu, Y., & Chen, Y. (2022). Association Between Familial Hypercholesterolemia and Risk of Cardiovascular Events and Death in Different Cohorts: A Meta-Analysis of 1.1 Million Subjects. Frontiers in Cardiovascular Medicine, 9. https://doi.org/10.3389/fcvm.2022.860196
Visual Sources
Comments