General Pathology Series: Inflammatory Cells Recruitment and Clearance
Foreword
Inflammation is a vital reaction mechanism to threats, and it is part of a beneficial defense system that has evolved and been conserved evolutionarily over thousands of years. But why is this process associated with diseases? While inflammation is primarily protective and helps maintain tissue equilibrium, uncontrolled inflammation can become detrimental, leading to the progression of chronic inflammatory diseases. This series aims to provide a comprehensive understanding of the inflammatory process by emphasizing its historical context, intricate mechanisms, and events that will determine its end or the development of diseases. Through six captivating chapters, readers will obtain a holistic perspective on inflammation and its significance in health and disease.
This series is divided into six articles including:
General Pathology Series: Inflammation - Historical Perspectives
General Pathology Series: Inflammatory Cells Recruitment and Clearance
General Pathology Series: Inflammatory Cells Recruitment and Clearance
The inflammatory process is characterized by the recognition of a foreign agent, which culminates with the release of inflammatory mediators and rapid changes in the microcirculation. These events allow the fast and effective recruitment of cells to the injured tissue to fight the foreign agent and repair the affected tissue. A substantial number of molecules (cytokines, chemokines, and inflammatory mediators) and different leukocytes (inflammatory cells) can initiate and contribute to the progression of the inflammatory process. These also include neutrophils, macrophages, eosinophils, and monocytes (Medzhitov, 2010). During the inflammatory process, there is an influx of cells (migration) into different compartments of the body (bone marrow, blood circulation, tissue, and organs), and their numbers can be rapidly changed in those tissues during an ongoing inflammatory process (George-Gay & Parker, 2003). The infiltration of inflammatory cells into tissues from the bloodstream is a fundamental aspect of inflammatory reactions, as these cells are primarily responsible for neutralizing harmful agents, eliminating bacteria, particles, and cellular debris, and assisting in tissue repair. Inflammatory cells can be produced and mature in a short period, as well as die and be removed (deCathelineau & Henson, 2003). All of these processes occur in an orchestrated manner, and abnormalities in these pathways can cause harmful effects for the host. In this chapter, we will delve into the events associated with the recruitment of immune cells to the inflammatory site and their subsequent removal from the affected tissue.
Inflammatory Cell Production in the Bone Marrow and Release in the Circulation
Cells present in the bone marrow, known as common progenitor cells and also referred to as hematopoietic stem cells, originate from erythroblasts (red blood cells), myeloblasts (progenitor of most white blood cells), and megakaryoblasts (progenitor of platelets) [Figure 1]. In simple terms, these stem cells differentiate and proliferate into red blood cells, neutrophils, monocytes, lymphocytes, eosinophils, and basophils. Then they are released into the blood circulation daily, being detected in absolute numbers by routine blood tests (George-Gay & Parker, 2003).

The production and differentiation of white blood cells (WBC) in the bone marrow and their release into the circulation are influenced by specific mediators that can be released locally or in the circulation, including colony-stimulating factors (e.g., granulocyte colony-stimulating factor [G-CSF], macrophage colony-stimulating factor [M-CSF], interleukins and tumor necrosis factor) (Wang, Erlacher, & Fernandez-Orth, 2022). Approximately 109 cells/kg body weight leave the bone marrow per day in healthy humans, and 50 to 75 percent of these cells are neutrophils (von Vietinghoff & Ley, 2008). The cells within the bone marrow compartment are classified into two populations: those that are in the process of maturation and those that are in a storage phase, awaiting to be released into the circulating pool. The storage of cells in the bone marrow allows a rapid response to the need for WBC activity, such as in inflammatory conditions or infections, making a two- to three-fold increase in circulating leukocytes possible in a few hours (von Vietinghoff & Ley, 2008). After the release in circulation, the estimated life span of a WBC depends on the cell type and its function and can vary from hours to days.
Complete Blood Count Test for Disease Diagnosis
A complete blood count, also called a CBC, is a simple blood test that is commonly required as part of a routine medical assessment. As the name suggests, it is a count of the different types of cells found in the blood. The CBC indicates the counts of white blood cells, red blood cells, and platelets, the concentration of hemoglobin, and the hematocrit (the percentage of red blood cell count in relation to the total volume of blood). The test can diagnose and monitor many different diseases, such as anemia, infection, inflammatory diseases, and cancer (Dean L., 2005). Results slightly outside the typical range on a CBC might not be of concern for someone who is healthy and has no symptoms of illness. However, results of a CBC outside the expected range might signal a need for additional testing or a visit to a specialist, since results above or below the typical range on complete blood count might point to health issues (George-Gay & Parker, 2003). Table 1 shows the expected normal range for complete blood count results for adults. The blood is measured in cells per liter (cells/L or cells/mm3) or grams per deciliter (grams/dL).
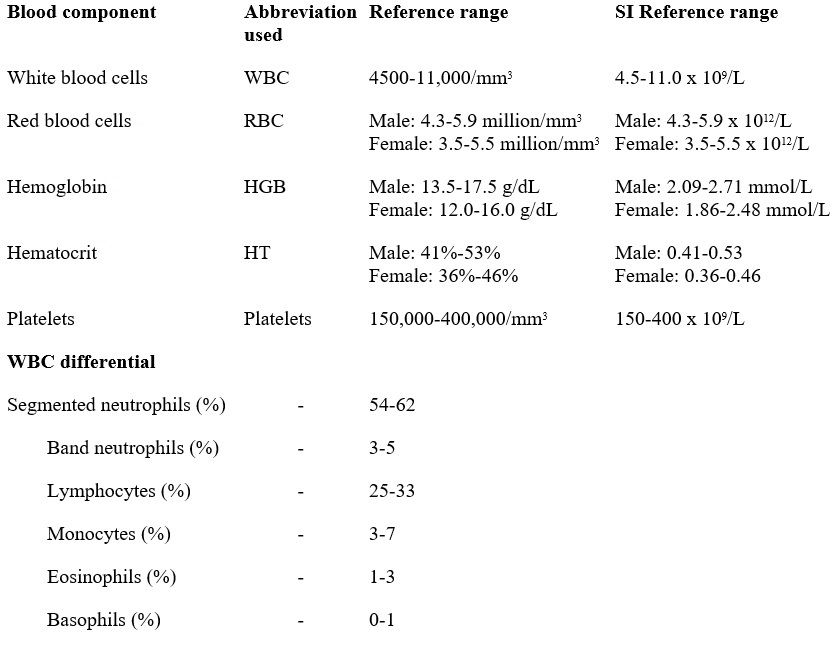
A low WBC count is known as leukopenia. Some conditions can cause bone marrow to fail to produce WBCs such as autoimmune disorders, radiation therapy, and chemotherapy, that destroy WBCs. Increased removal of WBCs from the circulation during infections as well as certain medicines and toxic substances can also drop WBC counts (George-Gay & Parker, 2003). Leukocytosis is a term used when the numbers of WBC count is higher than usual and it is most commonly due to an infection or inflammation. Immune system disorders, bone marrow diseases, reactions to medicines or foreign substances, hard exercise, and some types of cancers can increase WBC count (George-Gay & Parker, 2003). In addition to the total number of WBCs, it is also possible to evaluate the WBC differential counts (Table 1). Increased neutrophil count in the blood than the normal reference range is defined as neutrophilia, and it can be seen during infections, inflammation, and cancers. In situations of severe inflammation, it is possible to observe the presence of immature cells (band neutrophils) in the circulation by the WBC differential test. The increase of band neutrophils in blood is known as left shift, and the term is based on the shape of the cell nucleus during the stages of neutrophil maturation in the bone marrow (Honda, Uehara, Matsumoto, Arai, & Sugano, 2016) [Figure 2].
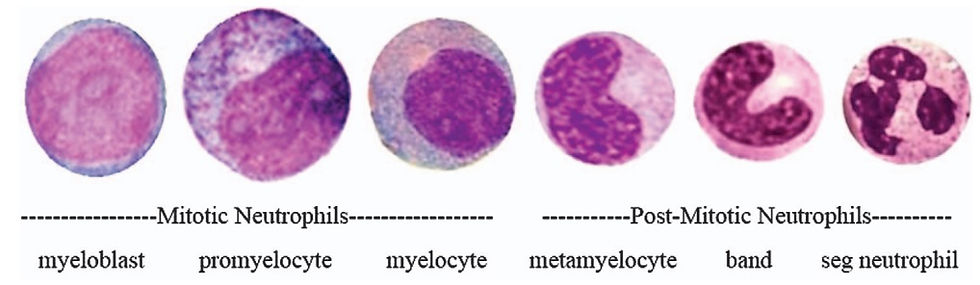
Furthermore, other WBCs can also be determined in a complete blood count test, including lymphocytes, monocytes, eosinophils, and basophils [Table 1]. For example, increased numbers of eosinophils (eosinophilia) in the blood are associated with allergic reactions and parasitic infections while high lymphocyte count (lymphocytosis) typically rises after infection conditions (George-Gay & Parker, 2003).
The results of red blood cell count, hemoglobin, and hematocrit are related since they each measure a feature of red blood cells. Lower than usual measures in these three areas are a sign of anemia. A red blood cell count that is higher than usual is known as erythrocytosis and could point to a medical condition such as blood cancer or heart disease. A platelet count that is lower than usual is known as thrombocytopenia, while a higher count than usual is known as thrombocytosis. Either can be a sign of numerous medical conditions that should be further investigated (George-Gay & Parker, 2003).
Inflammatory Cell Recruitment to Tissue
Once leukocytes reach the bloodstream, they can be recruited to tissues during an inflammatory response. This process is carefully regulated by a complex set of molecules produced and secreted in response to the inducing agent, which allows the interaction of circulating cells (leukocytes) with endothelial cells (blood vessel wall cells), enabling the migration of leukocytes from the circulation to the inflammatory site. After tissue damage, pro-inflammatory mediators and chemokines are released by resident cells (macrophages, dendritic cells, and epithelial cells) in the injured tissue, activating circulating leukocytes and inducing rapid changes in the adhesion properties between them and the endothelial cells of blood vessels (Simon & Green, 2005). Pro-inflammatory mediators “activate” the endothelium (a thin layer of endothelial cells) inducing the expression of adhesion molecules. In the lumen of the blood vessel, leukocytes first roll over the activated endothelium through weak selectin-glycoprotein interactions [Figure 3].

The rolling of leukocytes through the blood vessel allows the interaction of leukocytes with chemokines (chemoattractant molecules), resulting in the activation of integrins (Mac-1 and VLA) on the surface of the leukocytes, which display a higher affinity for the integrin ligands (ICAM and VCAM) on the endothelial cell surface (Yuan, Shen, Rigor, & Wu, 2012). Said interaction between integrins and integrin ligands leads to a tight adhesion and arrest of the leukocytes to the endothelium. After integrin-mediated crawling to an optimal emigration spot, the leukocytes transmigrate through the endothelial barrier into the surrounding tissue [Figure 3], where their migration will be guided further to the inflammatory site directed by the chemoattractant gradient (Nourshargh & Marelli-Berg, 2005). In a chemoattractant gradient, the concentration of chemokines is higher in one region but gradually decreases away from that region. Leukocytes can sense this concentration gradient by specific receptors and use it as a directional cue to move towards areas of higher chemokine concentration (Yuan et al., 2012).
The Importance of Phagocytosis and Efferocytosis at the Site of Inflammation
Once leukocytes are recruited to the site of inflammation, these cells will perform important functions to fight the harmful agent and reestablish tissue homeostasis. Among one of these functions, we can highlight phagocytosis. Phagocytosis is a cellular process characterized by the recognition and ingestion of particles (larger than 0.5 μm) mediated by a plasma membrane that forms a membrane-encased vesicle, known as a phagosome (Flannagan, Jaumouille, & Grinstein, 2012). This phagosome subsequently changes the structure of its membrane and the composition of its contents in a process known as phagosome maturation. The phagosome next fuses with lysosomes (organelles that contain an array of enzymes capable of breaking down molecules of different natures) to become a phagolysosome. This new organelle contains enzymes that can degrade the ingested particle efficiently (Uribe-Querol & Rosales, 2020) [Figure 4]. Only a specialized group of cells, called professional phagocytes, accomplish phagocytosis with high efficiency. Macrophages, neutrophils, monocytes, and dendritic cells, are some examples of professional phagocytes. At the site of inflammation, phagocytosis can contribute to the reorganization and renovation of the tissue and participate in the host defense eliminating microorganisms and foreign substances (Flannagan et al., 2012).
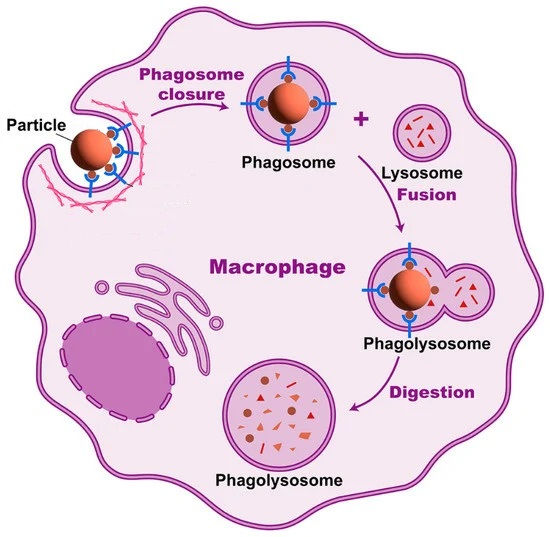
In the course of an inflammatory process, many tissue cells or recruited leukocytes may die. Cell death is a biological process that is necessary during the development, growth, and maintenance of tissues, but which can be crucial in immune responses. In the human body, billions of cells die per day constitutively via a process of programmed cell death called apoptosis. Apoptosis can be described morphologically by cell shrinkage and chromatin (DNA) condensation, followed by fragmentation of the entire cell forming apoptotic bodies, which are membrane-bound vesicles containing cellular components from the dying cell (Silva, 2010). These apoptotic bodies can be promptly cleared by phagocytes (especially macrophages and dendritic cells) and neighboring cells in a process that is termed efferocytosis (meaning “carrying to the grave”) (deCathelineau & Henson, 2003).
During a successful efferocytosis process, four important events can be observed [Figure 5].
Phagocyte recruitment: during the inflammatory response, “find me” signals are released. These signals are molecules released by apoptotic cells and help attract phagocytes to the inflammatory site.
Recognition: exposure of “eat me” signals, molecules are exposed on the surface of apoptotic cells (phosphatidylserine or calreticulin) and promote specific recognition by the phagocyte and subsequent internalization of apoptotic bodies - dead cells are removed before they lose their membrane integrity and its contents are released into the extracellular space preventing exposure of the tissue to toxic enzymes, oxidants, and other intracellular components.
Phagocytosis of apoptotic bodies: apoptotic bodies are ingested.
Digestion: ingested apoptotic bodies are processed by phagocytes, where the phagocytosed material goes through a series of maturation events, leading to its degradation within phagolysosomes (Lin et al., 2020).
After digestion within the phagolysosome, phagocytes produce anti-inflammatory cytokines (IL-10 and TGF-β) and pro-resolving mediators, which increase the efficiency of the efferocytosis process, induce resolution of inflammation, and also prevent the development of diseases (Kourtzelis, Hajishengallis, & Chavakis, 2020). In addition, “don’t eat me” signals are expressed in healthy cells, preventing efferocytosis, even when “eat me” signals are exposed in the microenvironment (Lin et al., 2020).

Defective removal of apoptotic cells resulting from impaired efferocytosis can lead to the perpetuation of inflammation and tissue dysfunction. When apoptotic cells are not cleared in an efficient and timely manner, they progress to a necrotic state known as secondary necrosis (Silva, 2010). Necrosis is a catastrophic form of cell death with loss of plasma membrane integrity and exposure of intracellular contents into the extracellular environment, leading to activation of immune response and consequently pro-inflammatory state [Figure 6]. Thus, efferocytosis represents an auxiliary process used by multicellular organisms as a strategy to avoid the deleterious consequences associated with the progression of apoptosis to secondary necrosis. In pathological conditions, neutrophil lysis due to secondary necrosis is particularly deleterious as these phagocytes are recruited in very high numbers to inflammatory sites and are extraordinarily rich in tissue-damaging cytotoxic molecules. A highly cytotoxic phagocyte molecule released by secondary necrotic cells is the neutrophil elastase, which is an important microbicidal molecule but may therefore perpetuate the inflammatory state, by inducing tissue damage and further neutrophil recruitment (Silva, 2010).

Consequences of Defective Clearance of Apoptotic Cells
Increasing evidence suggests that inadequate elimination of apoptotic cells, whether as a result of genetic abnormalities and/or a persistent disease state, contributes to the establishment and progression of several diseases. Studies suggested that if the organism fails to contain or clear the apoptotic cells and debris appropriately, tissue inflammation is prolonged and autoimmunity can ensue (Elliott & Ravichandran, 2010) [Figure 7].

Disorders such as systemic lupus erythematosus (SLE) have been associated with defects in tissue regeneration and impaired ability of leukocytes to recognize or eliminate cell debris. SLE is a chronic disease characterized by a dysregulated immune system that mistakenly attacks healthy tissues and organs. In animal studies in which efferocytosis is impaired, SLE-like autoimmunity develops. Less efficient macrophages and improper efferocytosis have been described in human SLE (Mahajan, Herrmann, & Munoz, 2016). The regulated nature of apoptotic cell death normally prevents leakage of intracellular contents. If, however, these cells are not removed in time, they undergo secondary necrosis leading to the exposure of intracellular antigens such as DNA, which can provoke a persistent systemic auto-inflammatory response, causing the tissue and organ damage observed in SLE. Although it is a multifactorial disease, both animal models and human research indicate a strong relationship between improper cell clearance and the development of SLE, representing the most studied example of autoimmune diseases in the context of defective efferocytosis (Mahajan et al., 2016).
While in SLE improper efferocytosis affects all tissues, inefficient apoptotic clearance in the lung was detected in several chronic inflammatory respiratory diseases, such as chronic obstructive pulmonary disease (COPD), cystic fibrosis, and asthma (Zheng, Abou Taka, & Heit, 2021). In these diseases, increased numbers of apoptotic cells have been reported in the lung tissue. Impaired efferocytosis has also been associated with the development of atherosclerosis. Atherosclerosis is a chronic vascular disease where the arteries become damaged, forming a plaque of fatty substances, cholesterol, cellular debris, calcium, and other elements, which can narrow and stiffen the affected arteries over time, increasing the risks for myocardial infarction and stroke (Yurdagul et al, 2017). After accumulating experimental evidence, it has demonstrated that impaired clearance of apoptotic cells is observed in atherosclerotic plaques, leading to the formation of an unstable necrotic core and the maintenance of inflammation (Yurdagul et al., 2017).
Defective efferocytosis and excessive accumulation of apoptotic cells have also been reported in numerous neurodegenerative diseases, such as Parkinson’s, Alzheimer’s, or Huntington’s diseases, as well as Multiple Sclerosis (Zhang et al, 2022). The central nervous system also requires efficient efferocytosis to achieve homeostasis by clearing the dying cells and preventing the spillover of proinflammatory and neurotoxic molecules. Research into the mechanisms of efferocytosis in the CNS is ongoing, and understanding this process may offer insights into potential therapeutic strategies for neurodegenerative diseases. Examples of other diseases associated with defective effects are included in Figure 8.

Case Study
A 25-year-old female patient seeks the emergency department because she feels abdominal pain, anorexia, nausea, and vomiting. The pain began 18 hours ago in the periumbilical region and is now localized to the right lower quadrant, accompanied by an episode of vomiting and a temperature of 38.3°C. The pain worsens with the contraction of the abdominal wall and when walking. Upon physical examination, the patient is prostrate. The complete blood count indicates leukocytosis (12,700/mm3) with a shift to the left—presence of 8% of band neutrophils and 70% of segmented neutrophils (Breeding & Conran, 2020).
The laboratory blood count test showed an increase in the total number of leukocytes (leukocytosis) with a shift to the left—8% of band neutrophils—, indicating the presence of immature blood cells in the peripheral blood. The most common cause of the appearance of left shift, associated with neutrophilia, is infections, especially bacterial infections. During a bacterial infection, large numbers of neutrophils are consumed in the early phase, stimulating the bone marrow to produce and release additional neutrophils. An acute bacterial infection usually depletes the normal reserves of mature neutrophils, and the bone marrow has to resort to releasing immature ones. The referenced case study is a typical case of acute appendicitis. Acute appendicitis is inflammation of the cecal appendix (a small, tubular, finger-like structure located near the junction of the small intestine, ileum, and the large intestine, cecum), caused, in the vast majority of cases, by obstruction of the lumen. With obstruction, there is a proliferation of microorganisms, mainly gram-negative bacteria and anaerobes, and infection of the appendix wall, which is the cause of most of the clinical signs of the syndrome that accompanies this disease. A young patient, like the one in this case study, without presenting comorbidities, can undergo an appendectomy (Breeding & Conran, 2020).
Conclusion
The inflammatory response is a finely regulated process that involves distinct cells and mediators that act in a coordinated manner to combat a threat and initiate the healing process. This orchestrated response involves changes in microcirculation, enabling inflammatory cells produced and matured in specific tissues to migrate to the affected areas where they combat infections. Over the years, understanding the dynamics of cell recruitment and migration has become crucial for diagnosing diseases, as alterations in circulating cell counts are often indicative of pathological conditions. These inflammatory cells migrate to the site of infection with the primary goal of neutralizing the harmful agents, through mechanisms such as phagocytosis. Typically, these cells undergo apoptosis. This prompts the activation of efferocytosis for the clearance of apoptotic cells and their debris. The efficient removal of inflammatory cells from the site of inflammation is vital for ensuring tissue restoration and repair. However, in certain pathological conditions, this clearance process may be ineffective, potentially leading to the onset and progression of diseases. Some examples of such diseases were mentioned here, where impaired cellular clearance mechanisms have been linked to their pathogenesis. Consequently, researchers are making efforts to unravel the intricate mechanisms governing cell recruitment and clearance, to develop innovative therapies for treating these conditions.
Bibliographical References
Breeding, E., & Conran, R. M. (2020). Educational Case: Acute Appendicitis. Academic Pathology, 7, 2374289520926640. https://doi.org/10.1177/2374289520926640
deCathelineau, A. M., & Henson, P. M. (2003). The final step in programmed cell death: phagocytes carry apoptotic cells to the grave. Essays in Biochemistry, 39, 105-117. https://doi.org/10.1042/bse0390105
Dean L. Blood Groups and Red Cell Antigens [Internet]. Bethesda (MD): National Center for Biotechnology Information (US); 2005. Available from: https://www.ncbi.nlm.nih.gov/books/NBK2261/
Elliott, M. R., & Ravichandran, K. S. (2010). Clearance of apoptotic cells: implications in health and disease. Journal of Cell Biology, 189(7), 1059-1070. https://doi.org/10.1083/jcb.201004096
Flannagan, R. S., Jaumouille, V., & Grinstein, S. (2012). The cell biology of phagocytosis. Annual Review of Pathology, 7, 61-98. https://doi.org/10.1146/annurev-pathol-011811-132445
George-Gay, B., & Parker, K. (2003). Understanding the complete blood count with differential. Journal of Perianesthesial Nursing, 18(2), 96-114; quiz 115-117. https://doi.org/10.1053/jpan.2003.50013
Honda, T., Uehara, T., Matsumoto, G., Arai, S., & Sugano, M. (2016). Neutrophil left shift and white blood cell count as markers of bacterial infection. Clinical Chimica Acta, 457, 46-53. https://doi.org/10.1016/j.cca.2016.03.017
Kourtzelis, I., Hajishengallis, G., & Chavakis, T. (2020). Phagocytosis of Apoptotic Cells in Resolution of Inflammation. Frontiers in Immunology, 11, 553. https://doi.org/10.3389/fimmu.2020.00553
Lin, D., Kang, X., Shen, L., Tu, S., Lenahan, C., Chen, Y., . . . Shao, A. (2020). Efferocytosis and Its Associated Cytokines: A Light on Non-tumor and Tumor Diseases? Molecular Therapy Oncolytics, 17, 394-407. https://doi.org/10.1016/j.omto.2020.04.010
Mahajan, A., Herrmann, M., & Munoz, L. E. (2016). Clearance Deficiency and Cell Death Pathways: A Model for the Pathogenesis of SLE. Frontiers in Immunology, 7, 35. https://doi.org/10.3389/fimmu.2016.00035
Medzhitov, R. (2010). Inflammation 2010: new adventures of an old flame. Cell, 140(6), 771-776. https://doi.org/10.1016/j.cell.2010.03.006
Nourshargh, S., & Marelli-Berg, F. M. (2005). Transmigration through venular walls: a key regulator of leukocyte phenotype and function. Trends in Immunology, 26(3), 157-165. https://doi.org/10.1016/j.it.2005.01.006
Silva, M. T. (2010). Secondary necrosis: the natural outcome of the complete apoptotic program. FEBS Letters, 584(22), 4491-4499. https://doi.org/10.1016/j.febslet.2010.10.046
Simon, S. I., & Green, C. E. (2005). Molecular mechanics and dynamics of leukocyte recruitment during inflammation. Annual Review of Biomedical Engineering, 7, 151-185. https://doi.org/10.1146/annurev.bioeng.7.060804.100423
Uribe-Querol, E., & Rosales, C. (2020). Phagocytosis: Our Current Understanding of a Universal Biological Process. Frontiers in Immunology, 11, 1066. https://doi.org/10.3389/fimmu.2020.01066
von Vietinghoff, S., & Ley, K. (2008). Homeostatic regulation of blood neutrophil counts. Journal of Immunology, 181(8), 5183-5188. doi:10.4049/jimmunol.181.8.5183
Wang, J., Erlacher, M., & Fernandez-Orth, J. (2022). The role of inflammation in hematopoiesis and bone marrow failure: What can we learn from mouse models? Frontiers in Immunology, 13, 951937. https://doi.org/10.3389/fimmu.2022.951937
Yuan, S. Y., Shen, Q., Rigor, R. R., & Wu, M. H. (2012). Neutrophil transmigration, focal adhesion kinase and endothelial barrier function. Microvascular Research, 83(1), 82-88. https://doi.org/10.1016/j.mvr.2011.06.015
Yurdagul, A., Jr., Doran, A. C., Cai, B., Fredman, G., & Tabas, I. A. (2017). Mechanisms and Consequences of Defective Efferocytosis in Atherosclerosis. Frontiers in Cardiovascular Medicine, 4, 86. https://doi.org/10.3389/fcvm.2017.00086
Zhang, Y., Wang, Y., Ding, J., & Liu, P. (2022). Efferocytosis in multisystem diseases (Review). Molecular Medicine Reports, 25(1). https://doi.org/10.3892/mmr.2021.12529
Zheng, D. J., Abou Taka, M., & Heit, B. (2021). Role of Apoptotic Cell Clearance in Pneumonia and Inflammatory Lung Disease. Pathogens, 10(2). https://doi.org/10.3390/pathogens10020134
Kommentare