Inside the Invisible World of Plasmids
Introduction
Imagine a world filled with invisible living things, each carrying within them a tiny circle of DNA. This is not the main set of DNA instructions, but an auxiliary one, providing unique survival tips and tricks. These tiny circles are called plasmids and they play an essential role in the microscopic universe of bacteria (Plasmids 101: What Is a Plasmid?, n.d.). Together with the main set of DNA instructions, they make up the genetic material of the bacteria.
Not only do they help bacteria thrive in challenging environments, but they also allow these minuscule organisms to share valuable survival information with each other, like tips on how to fight off the effects of antibiotics (Davies & Davies, 2010; Lederberg, 1952). But plasmids aren't just important to bacteria. They have also emerged as a crucial tool for scientists, allowing manipulation of genetic material for various applications, from creating life-saving medicines to modifying crops for improved yield and nutritional content (Cohen et al., 1973; Nelson, 2009).
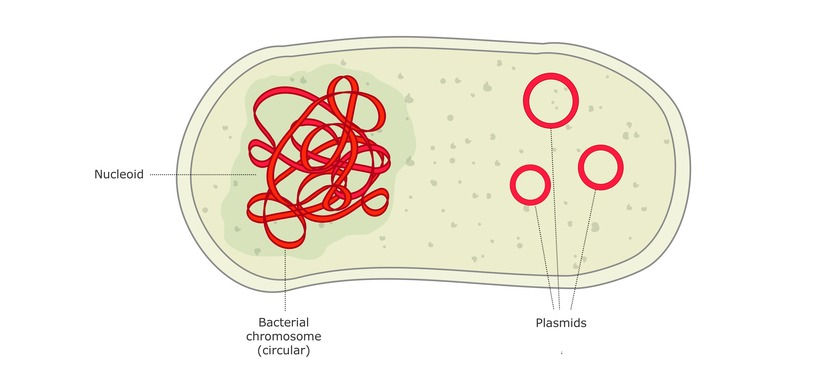
Despite their microscopic size, plasmids wield enormous power in their ability to affect life on both small and large scales. This article will delve into the fascinating universe of plasmids, exploring their role in bacterial survival and adaptation, their applications in biotechnology, and the ethical questions that arise from their usage.
Understanding Plasmids and their role in bacteria
Plasmids are unique elements in the world of microbiology. Think of them as a mini-instruction manual, made of DNA, that bacteria carry in addition to their main genetic material. This isn't a fixed part of the bacterial blueprint - instead, it can be swapped, copied, and even transferred between bacteria (Lederberg, 1952). One bacterium can pass its plasmid (and the special survival tips it contains) to another bacterium. This process, known as conjugation, helps spread beneficial traits, such as antibiotic resistance, across a bacterial community (Frost et al., 2005). Think of it as bacteria helping each other to better survive in challenging situations (Martínez, 2008). Plasmids typically contain a small number of genes, often less than 30, compared to thousands found in the bacterial chromosome (Couturier et al., 1988). However, these genes can provide a crucial survival advantage.
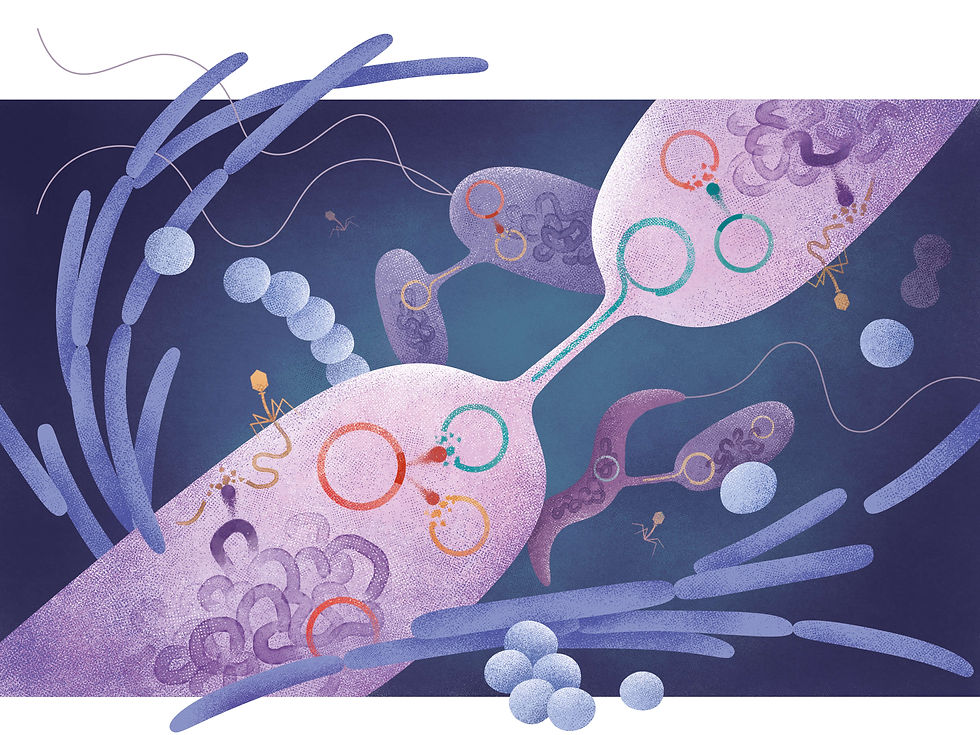
In the world of bacteria, the survival of the fittest is a harsh reality. With resources limited and competition high, the genes found on plasmids can make a crucial difference. For example, some plasmids carry genes for antibiotic resistance. When a bacterium carrying such a plasmid encounters an antibiotic, it can survive and continue to multiply, while other bacteria without this advantage may be wiped out (Davies & Davies, 2010).
Plasmids also have other survival tricks up their sleeve. Some contain genes that allow bacteria to digest substances they would otherwise find indigestible, essentially expanding their menu of potential food sources (Trevors, 1986). Others can even carry genes that transform a harmless bacterium into a potent pathogen, causing diseases in humans or other organisms (Couturier et al., 1988).
The Standard Structure of a Plasmid
In the realm of microbiology, plasmids are self-replicating DNA molecules that are usually circular and vary in size from one to over 200 kilobase pairs (kbp) (Sørensen et al., 2005). Despite their diversity, all plasmids share a basic structure, composed of a few critical regions.
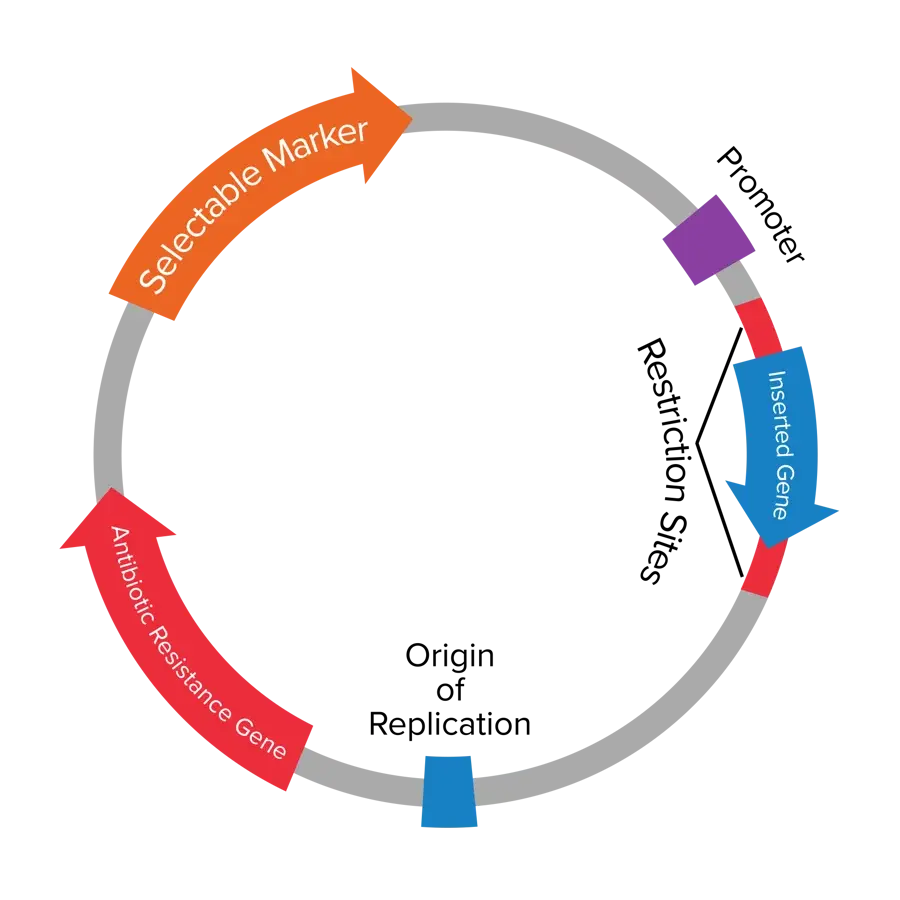
The primary part of a plasmid is the origin of replication (ori), a specific sequence of DNA where replication begins. Replication is a biological process during which the DNA makes an exact copy of itself. This process is vital to ensure that every new cell has the same genetic material as its parent. In the context of plasmids, the ori controls the initiation and regulation of this replication process, enabling the plasmid to be copied each time the bacterium divides (del Solar et al., 1998).
Another crucial region of a plasmid contains one or more genes that provide a specific trait or function to the bacterium, such as antibiotic resistance. These are the genes that can be transferred between bacteria or manipulated by scientists for various applications (Davies & Davies, 2010; Lederberg, 1952). Many plasmids also possess a so-called 'partitioning system' or 'par', which ensures fair distribution of plasmids during cell division. This system ensures that when a bacterium divides, each new cell receives at least one copy of the plasmid, thereby maintaining the plasmid in the population (Ebersbach & Gerdes, 2005).
Plasmids in Biotechnology
Biotechnology has unlocked a new realm of possibilities for plasmids. By harnessing the natural properties of these tiny DNA circles, scientists have been able to design tools and develop techniques that have revolutionized many aspects of our lives.
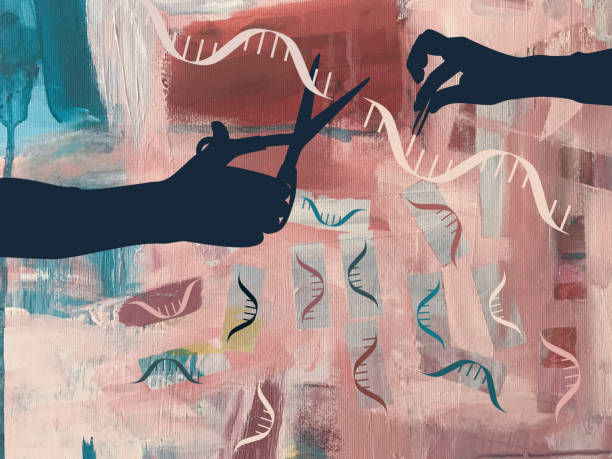
A key breakthrough in biotechnology came when scientists realized they could use plasmids as vectors - tools for carrying and introducing specific genes into a host organism (Cohen et al., 1973). By inserting a desired gene into a plasmid and introducing that plasmid into a bacterium, scientists could essentially turn the bacterium into a tiny factory, churning out the protein coded by the inserted gene.
One of the earliest and most profound applications of this technique was in the production of human insulin. Before this, people with diabetes who needed insulin injections relied on insulin extracted from pigs or cows, which could cause allergic reactions. However, by inserting the human insulin gene into a plasmid and introducing that plasmid into bacteria, scientists could make bacteria produce human insulin. This development not only ensured a reliable and safer supply of insulin but also made treatment more affordable for millions of people with diabetes worldwide (Walsh, 2005).
Plasmids have also been fundamental in the development of genetically modified (GM) crops. By inserting genes that confer desirable traits, like resistance to pests or tolerance to drought, scientists have been able to create crops that are more resilient and productive. For example, Bt-corn, a widely grown GM crop, contains a gene from a bacterium that produces a toxin lethal to many pests. This gene, inserted into corn plants using plasmids, has led to significantly reduced pesticide use and increased crop yields (Nelson, 2009).
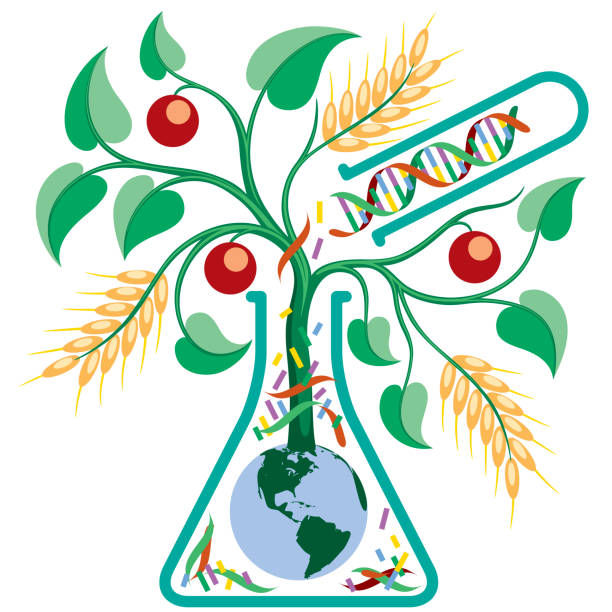
Beyond medicine and agriculture, plasmids are used in a variety of industrial applications. They are used to engineer bacteria or yeast to produce biofuels, enzymes for laundry detergents, and even bioplastics. By using renewable resources and biological processes, these techniques promise a more sustainable future for industries (Lee et al., 2019).
One example involves the use of plasmids to help bacteria digest oil spills. Certain species of bacteria, like Pseudomonas, naturally contain plasmids with genes that code for enzymes capable of breaking down hydrocarbons, the main component of oil. When there's an oil spill, these bacteria can increase in number and help break down the oil. However, their natural efficiency in doing this is limited (Van Hamme et al., 2003). Scientists have enhanced this process through the use of genetic engineering. By manipulating plasmids and inserting them into these bacteria, they have been able to enhance the bacteria's oil-degrading capabilities. In some cases, the engineered bacteria can also be more robust, allowing them to survive in harsh environments such as oil-contaminated waters where natural bacteria would struggle to thrive (Das & Chandran, 2011). This approach, while promising, does present its own challenges and requires stringent monitoring to ensure that the introduction of genetically engineered bacteria does not negatively impact local ecosystems. Nevertheless, it serves as an illustration of how plasmids and biotechnology can potentially provide solutions to serious environmental challenges.
Ethical Considerations and Regulatory Challenges
As with all forms of genetic engineering, the use of plasmids raises ethical and regulatory considerations. While the potential benefits of using genetically engineered organisms are significant, they must be weighed against potential risks and uncertainties.

One of the main concerns is the possibility of engineered genes escaping into the wild through horizontal gene transfer, potentially leading to unforeseen ecological consequences (Oye et al., 2014). For instance, if antibiotic resistance genes used in laboratory research were to escape into wild bacterial populations, it could contribute to the growing problem of antibiotic resistance (Nielsen et al., 1998). In agriculture, while GM crops have the potential to increase yield and reduce pesticide use, there are concerns about potential impacts on biodiversity, the emergence of pesticide-resistant pests, and the monopolistic control over seed resources by a handful of biotech companies (Failure to Yield: Evaluating the Performance of Genetically Engineered Crops | Union of Concerned Scientists, n.d.).
Moreover, as our understanding of genetics advances and technologies become more accessible, the potential for misuse increases, such as in the case of biohacking or bioterrorism (Kuiken, 2016). Regulations are in place in many countries to manage these risks, but there is an ongoing debate about the adequacy and effectiveness of these regulations (Marchant et al., 2009). Balancing the benefits and risks of plasmid use in biotechnology and ensuring an ethical and well-regulated approach to their use, will be critical to fully realizing their potential while protecting our ecosystems and societies.
Conclusion
In the quest to understand and harness the power of the microscopic world, plasmids have proven to be both an invaluable tool and an intriguing subject of study. These tiny loops of DNA, overlooked for so long in the shadow of the grandeur of the chromosomal DNA, are now recognized for their significant role in shaping bacterial communities and driving their adaptation to diverse environments.
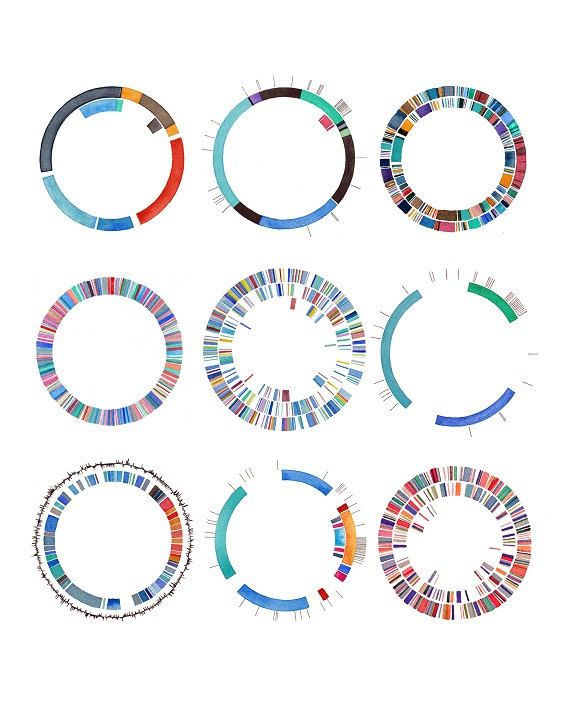
The versatility of plasmids, combined with their unique ability to transfer genes between organisms, has ushered in a new era in biotechnology. From the production of life-saving insulin to the development of crops resistant to pests and harsh environments, plasmids have transformed the approach to challenges in healthcare, agriculture, and environmental conservation.
However, like any powerful tool, the use of plasmids must be tempered by caution. The possibility of unintended ecological consequences, the potential for misuse, and the ethical questions raised by genetic engineering all highlight the need for robust regulatory frameworks and ongoing dialogue about how these technologies should be used.
In conclusion, while plasmids may be microscopic in size, their impact is undeniably vast. As society continues to explore their potential, it will be crucial to do so with an understanding of their complexities, a respect for their power, and a commitment to using them responsibly to benefit our world.
Bibliographic references
Cohen, S. N., Chang, A. C. Y., Boyer, H. W., & Helling, R. B. (1973). Construction of Biologically Functional Bacterial Plasmids In Vitro. Proceedings of the National Academy of Sciences, 70(11), 3240–3244. DOI: https://doi.org/10.1073/PNAS.70.11.3240
Couturier, M., Bex, F., Bergquist, P. L., & Maas, W. K. (1988). Identification and classification of bacterial plasmids. Microbiological Reviews, 52(3), 375–395. DOI: https://doi.org/10.1128/MR.52.3.375-395.1988
Das, N., & Chandran, P. (2011). Microbial Degradation of Petroleum Hydrocarbon Contaminants: An Overview. Biotechnology Research International, 2011, 1–13. DOI: https://doi.org/10.4061/2011/941810
del Solar, G., Giraldo, R., Ruiz-Echevarría, M. J., Espinosa, M., & Díaz-Orejas, R. (1998). Replication and control of circular bacterial plasmids. Microbiology and Molecular Biology Reviews : MMBR, 62(2), 434–464. DOI: https://doi.org/10.1128/MMBR.62.2.434-464.1998
Ebersbach, G., & Gerdes, K. (2005). Plasmid segregation mechanisms. Annual Review of Genetics, 39, 453–479. DOI: https://doi.org/10.1146/ANNUREV.GENET.38.072902.091252
Failure to Yield: Evaluating the Performance of Genetically Engineered Crops | Union of Concerned Scientists. (n.d.). Retrieved July 13, 2023, from https://www.ucsusa.org/resources/failure-yield-evaluating-performance-genetically-engineered-crops
Frost, L. S., Leplae, R., Summers, A. O., & Toussaint, A. (2005). Mobile genetic elements: the agents of open source evolution. Nature Reviews Microbiology 2005 3:9, 3(9), 722–732. DOI: https://doi.org/10.1038/nrmicro1235
Davies, J., & Davies, D. (2010). Origins and Evolution of Antibiotic Resistance. Microbiology and Molecular Biology Reviews, 74(3), 417–433. DOI: https://doi.org/10.1128/mmbr.00016-10
Kuiken, T. (2016). Governance: Learn from DIY biologists. Nature 2016 531:7593, 531(7593), 167–168. DOI: https://doi.org/10.1038/531167a
Lederberg, J. (1952). Cell Genetics and Hereditary Symbiosis., 32(4), 403–430. DOI: https://doi.org/10.1152/PHYSREV.1952.32.4.403
Lee, S. Y., Kim, H. U., Chae, T. U., Cho, J. S., Kim, J. W., Shin, J. H., Kim, D. I., Ko, Y. S., Jang, W. D., & Jang, Y. S. (2019). A comprehensive metabolic map for production of bio-based chemicals. Nature Catalysis 2019 2:1, 2(1), 18–33. DOI: https://doi.org/10.1038/s41929-018-0212-4
Marchant, G. E., Sylvester, D. J., & Abbott, K. W. (2009). What does the history of technology regulation teach us about nano oversight? The Journal of Law, Medicine & Ethics : A Journal of the American Society of Law, Medicine & Ethics, 37(4), 724–731. DOI: https://doi.org/10.1111/J.1748-720X.2009.00443.X
Martínez, J. L. (2008). Antibiotics and antibiotic resistance genes in natural environments. Science, 321(5887), 365–367. DOI: https://doi.org/10.1126/SCIENCE.1159483/SUPPL_FILE/365.MP3
Nelson, G. C. ; R. M. W. ; K. J. R. R. D. ; S. T. Z. T. R. C. M. S. P. A. B. M. M. M. V.-S. R. E. M. L. D. R. (2009). Climate change: Impact on agriculture and costs of adaptation. DOI: https://doi.org/10.2499/0896295354
Nielsen, K. M., Bones, A. M., Smalla, K., & van Elsas, J. D. (1998). Horizontal gene transfer from transgenic plants to terrestrial bacteria--a rare event? FEMS Microbiology Reviews, 22(2), 79–103. DOI: https://doi.org/10.1111/J.1574-6976.1998.TB00362.X
Oye, K. A., Esvelt, K., Appleton, E., Catteruccia, F., Church, G., Kuiken, T., Lightfoot, S. B. Y., McNamara, J., Smidler, A., & Collins, J. P. (2014). Regulating gene drives. Science, 345(6197), 626–628. DOI: https://doi.org/10.1126/SCIENCE.1254287/SUPPL_FILE/1254287.MP3
Plasmids 101: What is a plasmid? (n.d.). Retrieved July 13, 2023, from https://blog.addgene.org/plasmids-101-what-is-a-plasmid
Sørensen, S. J., Bailey, M., Hansen, L. H., Kroer, N., & Wuertz, S. (2005). Studying plasmid horizontal transfer in situ: a critical review. Nature Reviews. Microbiology, 3(9), 700–710. DOI: https://doi.org/10.1038/NRMICRO1232
Trevors, J. T. (1986). Plasmid curing in bacteria. FEMS Microbiology Letters, 32(3–4), 149–157. DOI: https://doi.org/10.1016/0378-1097(86)90286-7
Van Hamme, J. D., Singh, A., & Ward, O. P. (2003). Recent Advances in Petroleum Microbiology. Microbiology and Molecular Biology Reviews, 67(4), 503–549. DOI: https://doi.org/10.1128/MMBR.67.4.503-549.2003/ASSET/17CE8F2C-3420-4D11-B5D3-70A3A63B0AF2/ASSETS/GRAPHIC/MR0430023004.JPEG
Walsh, G. (2005). Therapeutic insulins and their large-scale manufacture. Applied Microbiology and Biotechnology, 67(2), 151–159. DOI: https://doi.org/10.1007/S00253-004-1809-X/METRICS
Comments