Mechanisms of Developmental Biology Series: Journey to Life
- Gülce Tekin
- Nov 26, 2023
- 18 min read
Updated: Sep 11, 2024
Foreword
The progression from a single-cell entity to a multicellular organism entails a series of intricate stages. This intricate journey involves the orchestrated activities of stem cells and the process of differentiation, which collectively constitute the life cycle of complex, multicellular organisms. The domain of developmental biology transcends human boundaries, encompassing studies of animals and plants alike, underscoring its extensive purview. The pursuit of unraveling the intricate mechanisms governing organismal development not only illuminates the origins of life but also provides invaluable insights into the molecular and cellular underpinnings of development across diverse species. The curriculum of this developmental biology series serves as an illuminating beacon, shedding light on the myriad manifestations of life on our planet. To gain a more profound comprehension of developmental processes, this series will traverse multiple interdisciplinary avenues, including embryology, medical science, and evolutionary biology. Through this holistic approach, we endeavor to uncover the underlying principles governing the diverse tapestry of life forms on Earth.
This series will be divided into the following sections: 1. Mechanisms of Developmental Biology Series: Essence of All
2. Mechanisms of Developmental Biology Series: How It All Begins
3. Mechanisms of Developmental Biology Series: Journey to Life
4. Mechanisms of Developmental Biology Series: Offshoot of a Plant
5. Mechanisms of Developmental Biology Series: Epagoge in Development: From Embryology to Evolution
6. Mechanisms of Developmental Biology Series: Cutting-edge Techniques in Developmental Biology
Mechanisms of Developmental Biology Series: Journey to Life
In the process of fetal growth, following organogenesis, the embryo enters the fetal stage characterized by substantial size and mass increase. The placenta plays a pivotal role in modulating fetal growth, while tissue differentiation and maturation involve the orchestrated expression of specific genes, ensuring the formation and maintenance of tissues and organs. The four fundamental tissue types—epithelial, connective, muscle, and nervous—collaborate to create organs and systems, showcasing the body's complexity. Connective tissue, diverse in types such as loose and dense, provides structural support. Neurons, integral to the nervous system, undergo phased development, creating intricate networks vital for bodily functions. Skeletal muscle development involves myoblasts- skeletal muscle precursor cells- originating from the mesoderm, contributing to muscle fiber formation. The circulatory system, crucial for nutrient supply, undergoes early development, with the heart, vessels, and specialized adaptations ensuring embryonic nourishment. Immune system development begins in the yolk sac and progresses through organs like the liver, spleen, and thymus, shaping the foundation for immune response. Sensory organ development, including olfactory, gustatory, visual, and auditory systems involves unique structures arising from ectodermal thickenings or branchial arches. Sexual differentiation hinges on sex chromosomes, initiating intricate processes in gonadal and genital development, resulting in the formation of male or female reproductive structures. All the systems come together and integrate to form a fully developed and functional organism. The complexity of fetal growth involves the precise coordination of numerous physiological processes, ensuring that organs and systems work harmoniously to support life. As the embryo progresses through the fetal stage, the culmination of tissue differentiation, organ maturation, and system integration results in the formation of a sophisticated and intricate organism. The interplay of genetic programs, cellular differentiation, and the influence of environmental factors ensures the harmonious growth and maturation of the fetus, setting the stage for a healthy and fully developed individual.
Fetal Growth
After organogenesis, which is the process of organ formation during embryonic development, the next stages in development involve further growth and maturation of the embryo, and specialization of the organs and tissues. With the major organs being formed during organogenesis, the developing organism enters the fetal stage in which there is a substantial increase in size and mass. The fetus undergoes rapid growth, and various body structures, such as limbs, head, and trunk continue to develop and take on their characteristic proportions. The growth of the fetus is highly modulated by the activity of the placenta, supporting the growth and development of the fetus. The placenta is a vital organ formed during embryogenesis and plays a crucial role in supporting the growth and development of the fetus. It acts as an interface between the maternal and fetal circulatory systems, facilitating the exchange of nutrients, oxygen, and waste products. Trophoblast cells invade the uterine lining, a process known as trophoblast invasion which is essential for securing the placenta to the uterine wall, establishing a stable connection for nutrient and gas exchange. The proper invasion of trophoblast cells and the remodeling of maternal blood vessels are crucial for establishing optimal uteroplacental blood flow. If this process is disrupted, as seen in conditions like pre-eclampsia, it can lead to complications such as reduced blood flow to the placenta, impacting fetal growth and development (Mayer, 2013). Early identification and management of these conditions are essential to mitigate potential risks to both the mother and the developing fetus.
Tissue Differentiation and Maturation
Tissues consist of various cell types that work together to carry out specific functions. Cells in different tissues and organs continue to differentiate into specialized cell types as the fetus continues to grow. This process involves the expression of specific genes that determine the function and characteristics of each cell. For example, in the endothelial tissue lining of the gastrointestinal tract, different cell types perform distinct tasks. Some cells are responsible for nutrient absorption, while others, such as goblet cells, secrete mucus to facilitate the smooth movement of digestive contents. Transcription factors, proteins that bind to DNA and regulate the transcription of specific genes, and regulatory elements control the expression of specific genes, ensuring that each cell type produces the necessary proteins for its specialized role within the tissue (Wragg et al., 2020). The coordinated differentiation of various cell types is crucial for the formation and maintenance of tissues and organs. Through specific signaling cues, transcriptional regulation, and epigenetic modifications, cells adopt distinct fates that contribute to the complexity and functionality of the overall organism.
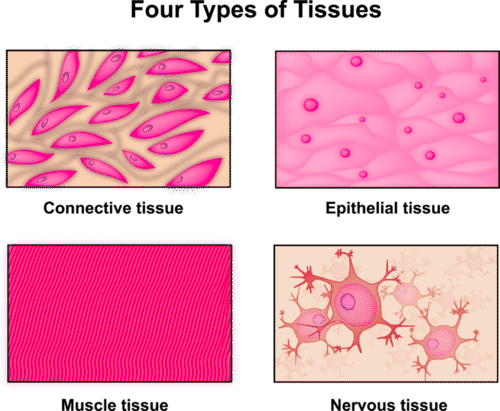
There are four basic types of tissue in the bodies of all animals, including the human body: epithelial, connective, muscle, and nervous tissues. Epithelial tissue is composed of closely packed cells that form a protective layer covering body surfaces or lining various organs and cavities. It serves functions such as protection, absorption, secretion, and sensation. The skin's outer layer (epidermis) and the lining of the digestive tract can be given as examples of this tissue type. Connective tissue provides support, structure, and connectivity to different parts of the body and consists of cells dispersed within an extracellular matrix that may contain fibers, ground substance, and fluid. Connective tissues include bone, blood, cartilage, adipose tissue, and tendons, among others. Muscle tissue is responsible for contraction and movement, and there are three main types of muscle tissue: skeletal muscle (attached to bones and responsible for voluntary movement), smooth muscle (found in the walls of internal organs and responsible for involuntary movements), and cardiac muscle (found in the heart and responsible for pumping blood). Nervous tissue is specialized for communication and control. It consists of neurons, which transmit electrical impulses, and glial cells, which provide support and protection to neurons. Nervous tissue makes up the brain, spinal cord, and peripheral nerves, playing a crucial role in the coordination of body functions and responses to environmental stimuli (Farley et al., 2012). These tissue types work together to form organs, structures, and systems in the body, contributing to the overall function and integrity of the organism. Organs are composed of multiple tissues organized into specific structures, and these organs, in turn, function together as systems within the body. Understanding the characteristics and functions of these fundamental tissue types is essential to comprehend the complexity of the human body and other animals.
Connective Tissue Formation
Connective tissue is a term used to describe a diverse group of tissues that play a crucial role in providing structural support, connecting and binding tissues, and contributing to the overall integrity and function of various organs and systems in the body. Connective tissue is characterized by the presence of cells, fibers, and a ground substance and it includes both loose and dense connective tissue. Loose connective tissue is characterized by a more open framework. It includes areolar tissue, which is found beneath the skin and surrounds organs, providing support and elasticity. On the other hand, dense connective tissue is more tightly packed and contains a higher proportion of collagen fibers, providing strength and support. Dense regular connective tissue, found in tendons and ligaments, has collagen fibers arranged in parallel, conferring resistance to pulling forces. Dense irregular connective tissue, found in the skin's dermis, has collagen fibers arranged in a random pattern, providing strength in multiple directions (Kamrani et al., 2023).
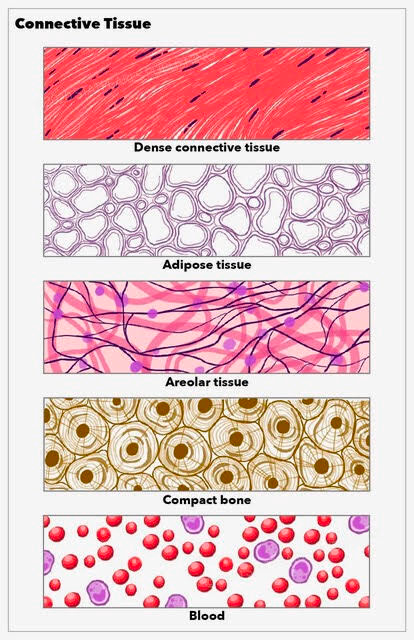
Specialized connective tissues include adipose tissue, cartilage, bone tissue, blood, and reticular tissue. Adipose tissue primarily stores fat and acts as an energy reservoir, an insulator, and provides cushioning and protection to organs. Cartilage tissue is firm and flexible with a gel-like ground substance. It provides support, cushioning, and a smooth surface for joint movement. Types of cartilage include hyaline cartilage (found in the nose and trachea), elastic cartilage (found in the ear), and fibrocartilage (found in intervertebral discs). Bone tissue is characterized by its hard, mineralized extracellular matrix. It provides support, and protection, and facilitates mineral storage and blood cell formation in the bone marrow. Blood is a specialized connective tissue composed of blood cells (red and white) suspended in a fluid matrix called plasma. It plays a crucial role in transportation, immune defense, and maintaining homeostasis (Nezwek & Varacallo, 2022). Lastly, reticular tissue forms a delicate network of fibers that supports various organs, such as the spleen and lymph nodes by providing structural support and a framework for cells.
Neuronal Development
Neurons are among the oldest and most specialized cell types in the animal kingdom. The most notable feature is their elongated shape, with a long axon that serves to transmit electrical signals over long distances and branching dendrites that receive signals from other neurons, creating a network of communication. The development of the nervous system unfolds in distinct phases with unique processes and challenges that involve the origin and differentiation of various neural components, the growth of axons and dendrites to establish provisional connections, and the ongoing refinement of these connections through interactions guided by electrical signals. Neurons communicate with each other through specialized junctions called synapses and the formation of synapses is a critical aspect of neural development. Neurons must selectively synapse with specific target cells to create a functional network through a process that involves the recognition of molecular cues and the establishment of precise connections (Alberts et al., 2022). One of the central challenges in neural development is to understand how axons and dendrites grow, navigate through the developing tissue, and establish connections with specific target cells. The growth of these processes is highly regulated, involving guidance cues and molecular signals that direct their pathfinding.
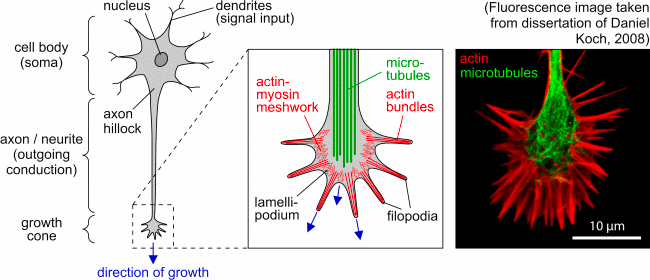
In the early stages of neural development, various components of the nervous system—neurons, glial cells, sensory cells, and muscles—originate from separate locations in the embryo. Initially, these components are unconnected and during this phase, each component follows its own local developmental program. "Neurons are born and acquire specific characteristics based on the place and time of their birth by a process that is regulated by inductive signals and gene regulatory mechanisms, similar to those observed in the development of other tissues. In the second phase of neural development, a process called morphogenesis occurs, specifically in the nervous system. Axons and dendrites, the processes of neurons responsible for transmitting signals, grow out along specific routes, setting up a provisional but orderly network of connections between the initially separate parts of the nervous system" (Alberts et al., 2022).
The guidance of axon and dendrite growth involves molecular cues and signaling mechanisms that direct their navigation through the developing tissue. The final phase of neural development extends into adult life and involves the refinement of connections within the nervous system by interactions among the widely dispersed components of the system, depending on the electrical signals that pass between neurons (de Vellis et al., 1999). As connections are established and neural circuits begin to function, electrical signals play a critical role in adjusting and fine-tuning the strength and specificity of these connections. This ongoing process of refinement continues throughout an individual's life, contributing to learning, memory, and adaptive responses to the environment.
Muscle Development
Myoblasts originate from the mesodermal layer during embryonic development, proliferate and differentiate into multinucleated myotubes, and form muscle fibers (Velleman, 2007). They fuse, contributing their nuclei to the growing myotubes which are the early structural units of muscle fibers. So, myotube formation contributes to the formation of mature muscle fibers, which is a crucial process for the development and growth of skeletal muscles. Primary myogenesis occurs during the embryonic stage, resulting in the formation of primary muscle fibers. While primary myofibers are formed during the embryonic stage, their impact on the subsequent size and number of muscle fibers is relatively minor. Secondary myogenesis takes place during the fetal stage, leading to the development of secondary muscle fibers that count for the majority of muscle fiber formation. Maternal nutrition significantly affects skeletal muscle development during the fetal stage due to its influence on myogenic progenitor cell proliferation (Yan et al., 2013).
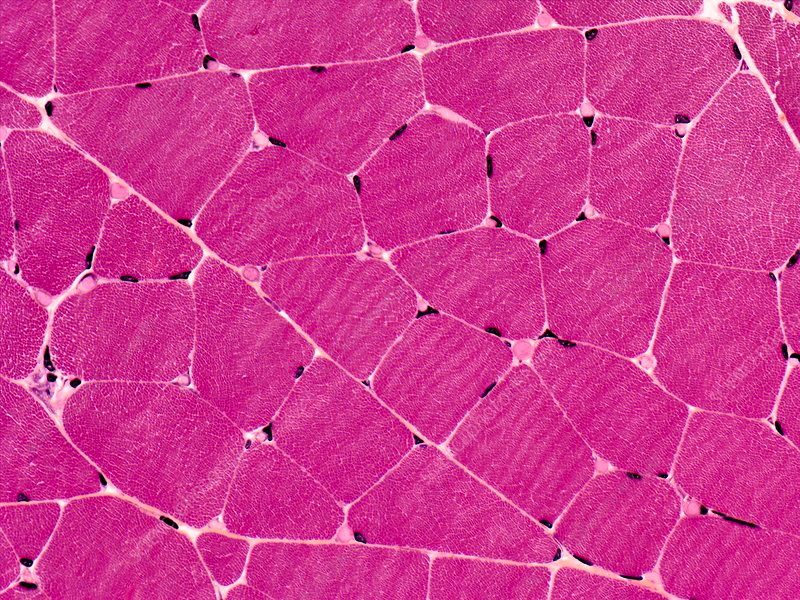
Postnatal development involves an increase in muscle fiber size rather than the net formation of new muscle fibers, mostly via satellite cells, located between the sarcolemma and basal lamina. Upon activation, satellite cells undergo asymmetric proliferation, with some cells differentiating into myoblasts. These myoblasts fuse with existing muscle fibers, contributing to increased fiber size and the incorporation of new nuclei (Yan et al., 2013). Recent studies suggest that satellite cells may have the capacity to differentiate into cell types other than muscle cells, such as adipocytes and fibroblasts.
Circulatory System Development
Oxygen and metabolite supply is crucial for the development of the circulatory system. In the early stages of embryo development, the process of diffusion is sufficient to provide oxygen and metabolites. As the embryo grows, diffusion becomes limited, prompting the development of the circulatory system to ensure an efficient supply of essential nutrients. So, the circulatory system becomes functional early in development, often before other organs and systems are fully formed. The heart originates from the mesoderm, a middle embryonic tissue layer. Initially, the heart is a tube that forms connections with blood vessels also developing in the mesoderm while surrounding mesodermal cells create the myocardium, the muscular wall of the heart. The heart is enclosed in the pericardial coelom, a dedicated section of the body cavity separated by partitions (Mathew & Bordoni, 2023). Over time, the heart tube bends, grows, and undergoes septation to form distinct chambers.

Vasculogenesis serves as the biological mechanism that addresses the need for a circulatory system by creating essential vascular structures. Similarly, angiogenesis encompasses the formation of neovessels from pre-existing vessels, contributing to the growth and expansion of the circulatory network as the organism develops. Once vessels are formed, remodeling occurs to refine their structure based on metabolic requirements and tissue-specific constraints, ensuring that the circulatory system aligns with the evolving needs of developing organs and tissues. These circulatory modifications ensure oxygenated blood is directed from external sources to nourish the developing embryo. In mammals, blood from the placenta follows a specific route, bypassing the lungs until birth. The placenta serves as a vital organ for nutrient and gas exchange during pregnancy (Udan et al., 2013). Fetal circulation involves specialized shunts that ensure proper blood flow, compensating for the nonfunctional fetal lungs and adapting to the unique conditions of life. These adaptations ensure that the embryo receives oxygen and nutrients essential for its development.
Immune System Development
The human immune system undergoes a long journey of development during pregnancy, shaping the foundation for a functional immune response in newborns. This process begins around the third week of gestation with primitive hematopoiesis in the yolk sac, where mesodermal cells transform into hematopoietic cells, marking the start of the first wave of blood cell production. By the fourth week, the yolk sac becomes a hub for various immune cell types, including hematopoietic stem cells, natural killer cell progenitors, and mast cells. These cells play crucial roles in the developing embryo, contributing to essential functions such as allergic responses. Additionally, the yolk sac gives rise to macrophages, which later populate vital organs like the brain, liver, lungs, and skin (Hossein et al., 2022). Around the same time, in the aorta-gonad-mesonephros (AGM) region, clusters of hematopoietic cells are called intra-aortic hematopoietic cluster (IAHC) cells. IAHCs emerge, contributing to the formation of hematopoietic stem cells. The AGM region becomes a significant site for hematopoiesis, ensuring the development of various blood cell lineages.

As the embryo development progresses, the liver, originating from the embryonic gut floor, becomes instrumental in hematopoietic differentiation by hosting macrophages, monocytes, dendritic cells, and B cells. The bone marrow, vital for B cell development, is established, further contributing to the maturation of immune cells. The spleen, a crucial organ for immune function, contributes to the production of both red and white blood cells in the second trimester. Simultaneously, the thymus, responsible for T cell development, starts forming from the third pharyngeal pouch. Lymph nodes, essential for immune surveillance, appear in various regions as gestation progresses, playing a critical role in monitoring and responding to pathogens (Simon et al., 2015). The development of T lymphocytes, primarily in the thymus, initiates at eight weeks, with regulatory T cells appearing around 12–14 weeks. B lymphocyte development involves the production of various B cell types, with a higher percentage of CD5+ B cells observed in fetuses. Immunoglobulin production starts early, with significant amounts of IgG and IgM—glycoprotein-based antibodies produced by the immune system in response to the presence of foreign substances—produced by the spleen. Serum IgG levels dramatically increase until birth, facilitated by placental transfer (Hossein et al., 2022).
Sensory Organ Development
The development of sensory organs involves specialized thickenings in the ectodermal layer known as placodes. Placodes are unique developmental entities that give rise to distinct sensory structures and are indispensable for the overall development and functionality of various organ systems (Francis-West et al., 2002). The olfactory organ, responsible for the sense of smell, emerges as paired thickenings of the ectoderm near the head's tip, evolving into olfactory pits and then sacs. Within these sacs, a specialized area becomes olfactory in function, hosting spindle-shaped olfactory cells that project receptive olfactory hairs beyond the epithelium's free surface, forming a connection with the brain through nerve fibers. This phenomenon highlights the precision required for the establishment of sensory perception.

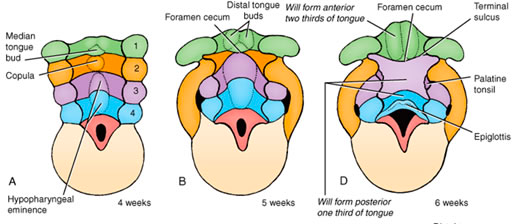
Gustatory organs, associated with taste, primarily arise on the tongue, manifesting as taste buds on lingual papillae. Each taste bud, a barrel-shaped specialization within the epithelium, comprises tall cells, some differentiating into taste cells with receptive gustatory hairs (Majoris et al., 2021). Sensory nerve fibers terminate at the surface of these cells, while other tall cells serve supportive functions. The formation of taste buds forms the coordination necessary for the development of the sense of taste.
The eye, a complex structure enabling vision, begins as shallow grooves on the forebrain's sides, swiftly forming indented optic cups connected to the brain by optic stalks. The optic cup differentiates into the retina, ciliary body, and iris. The retina's neural layer undergoes intricate layering, with visual cells bearing photoreceptive rods or cones, bipolar cells, and ganglion cells forming axons that connect with the brain. Simultaneously, the lens arises as an ectodermal thickening, forming a vesicle that detaches, giving rise to transparent lens fibers. The mesoderm forms the sclera, choroid, and iris. The eyelids, originating from adjacent skin folds, complete the eye's structural development (Heavner & Pevni, 2012).
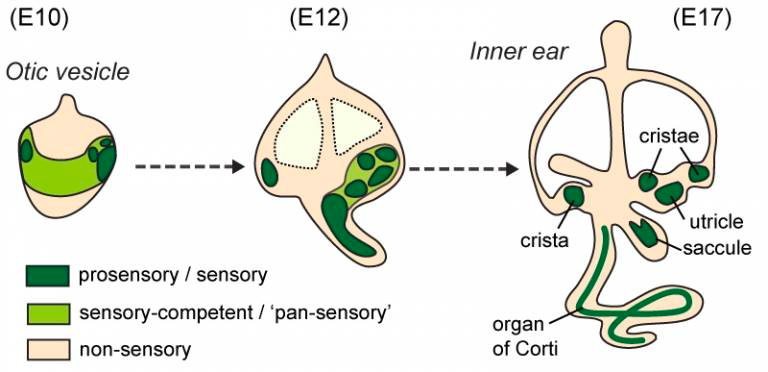

The formation of the ear, responsible for auditory and vestibular senses, starts from the emergence of the auricle from hillocks on the first and second branchial arches, while the external auditory canal forms from the deepening ectodermal groove between these arches. The auditory tube and tympanic cavity expand from the endodermal pouch between the arches, and the eardrum's future site emerges where the ectodermal groove and endodermal pouch meet. The internal ear's epithelium begins as an ectodermal thickening, forming the otocyst, which differentiates into the cochlear duct for hearing and chambers (utricle and saccule) related to balance. The otocyst's dorsal part remodels into semicircular ducts, essential for detecting movement, with fibers of the acoustic nerve growing among specialized receptive cells in these divisions.
Sexual Differentiation
Sexual differentiation in humans is a complex and highly coordinated process that begins with the determination of biological sex by the complement of sex chromosomes in an undifferentiated embryo. Initially, the conceptus is distinguishable by its karyotype, with males possessing XY chromosomes and females having XX chromosomes. After this initial determination, a dynamic and intricate process called sex differentiation unfolds, regulating the development of the indifferent gonad, internal genitalia, and external genitalia along either a male or female path (Aatsha et al., 2023).
In males (46 XY), the presence of the SRY gene triggers gonadal differentiation into testes. The SRY gene encodes the sex-determining region Y protein (SRY protein), also known as the testis-determining factor (TDF) that facilitates the differentiation of Sertoli cells, leading to the production of Müllerian inhibiting substance (MIS) or anti-Müllerian hormone (AMH). These substances play a crucial role in the regression of Müllerian ducts, leading to the formation of male internal genitalia. The testes further develop by recruiting vasculature through a remodeling mechanism. Conversely, in females (46 XX), the absence of an active SRY gene allows ovarian development, involving the growth of gonadal cords into the medulla of the developing ovary (Aatsha et al., 2023). This process forms irregular cell clusters, later replaced by vascular stroma and the differentiation of cortical cords from the surface epithelium. The primordial germ cells differentiate into oogonia, initiating the process of folliculogenesis. The ovary remains histologically indistinct until approximately 10 weeks post-fertilization.
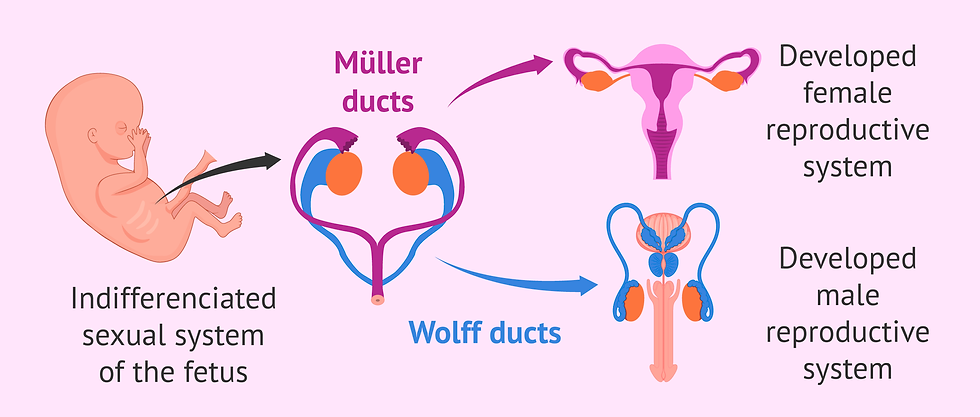
External genitalia, initially indifferent, undergo sex-specific differentiation. In males, dihydrotestosterone (DHT) induces the growth and elongation of the genital tubercle, forming the penis and scrotum. In females, the absence of androgens allows the genital tubercle to enlarge and form the clitoris. The labioscrotal folds remain largely unfused in females, forming the labia majora, while the urogenital folds give rise to the labia minora. Vaginal development involves the Müllerian ducts and the urogenital sinus, resulting in the formation of the sinovaginal bulbs, which fuse to create the vaginal plate (Rey et al., 2020). This plate develops into the vaginal canal, with the hymen separating it from the urogenital sinus. Besides the healthy development patterns, a range of variations in sex characteristics is also possible in some individuals whose reproductive anatomy does not follow the definitions of male or female as mentioned above. These variations may include "chromosomes (XX, XY, XXY, etc.), gonads, hormones, or genitalia" that differ from the typical male or female patterns (Griffiths, 2018). An interesting case can be "hermaphroditism" where the presence of both male and female reproductive organs can be observed in the same individual. This condition is relatively rare in humans, but it does occur in some animals and plants.
Conclusion
The journey of embryonic development is a marvel of coordination, and precision, where a single fertilized cell transforms into a complex, functioning organism. The stages of fetal growth, tissue differentiation, and organ maturation are carefully regulated, with the placenta and various developmental mechanisms playing crucial roles. The collaboration of diverse tissue types, the formation of intricate neural networks, the development of skeletal muscles, and the establishment of vital systems, such as the circulatory and immune systems, all contribute to the emergence of a fully-formed organism. The sensory organs, instrumental for perception, and the intricate process of sexual differentiation add further layers to the narrative of embryonic development. This journey, from the earliest stages to the formation of distinct organs and systems, underscores the remarkable moments of life's inception and emphasizes the significance of understanding these processes for both scientific knowledge and medical advancement. Insights into embryonic development have far-reaching implications, informing fields such as reproductive medicine, genetics, and regenerative medicine. Unmasking the mysteries of life empowers researchers and healthcare professionals to address developmental disorders, optimize infertility treatments, and explore innovative therapies.
Bibliographical References
Alberts, B., Johnson, A., Lewis, J., et al. (2002). Molecular Biology of the Cell. 4th edition. New York: Garland Science. Neural Development. Available from: https://www.ncbi.nlm.nih.gov/books/NBK26814/
Aatsha, P.A., Arbor, T.C. & Krishan, K. (2023). Embryology, Sexual Development. In: StatPearls [Internet]. Treasure Island (FL): StatPearls Publishing. Available from: https://www.ncbi.nlm.nih.gov/books/NBK557601/
de Vellis, J., & Carpenter, E. General Development of the Nervous System. In: Siegel GJ, Agranoff BW, Albers RW. (1999). Basic Neurochemistry: Molecular, Cellular and Medical Aspects. 6th edition. Philadelphia: Lippincott-Raven; Available from: https://www.ncbi.nlm.nih.gov/books/NBK28253/
Farley, A., McLafferty, E., & Hendry, C. (2012). Cells, tissues, organs and systems. Nursing standard (Royal College of Nursing (Great Britain) : 1987), 26(52), 40–45. https://doi.org/10.7748/ns2012.08.26.52.40.c9248
Francis-West, P. H., Ladher, R. K., & Schoenwolf, G. C. (2002). Development of the sensory organs. Science progress, 85(Pt 2), 151–173. https://doi.org/10.3184/003685002783238852
Griffiths, D. A. (2018). Shifting syndromes: Sex chromosome variations and intersex classifications. Social studies of science, 48(1), 125–148. https://doi.org/10.1177/0306312718757081
Heavner, W., & Pevny, L. (2012). Eye development and retinogenesis. Cold Spring Harbor perspectives in biology, 4(12), a008391. https://doi.org/10.1101/cshperspect.a008391
Hossain, Z., Reza, A.H.M.M. & Qasem, W.A. (2022). Development of the immune system in the human embryo. Pediatr Res 92, 951–955 . https://doi.org/10.1038/s41390-022-01940-0
Kamrani, P., Marston, G. & Arbor, T.C. (2023). Anatomy, Connective Tissue. In: StatPearls [Internet]. Treasure Island (FL): StatPearls Publishing. Available from: https://www.ncbi.nlm.nih.gov/books/NBK538534/
Majoris, J.E., Foretich, M.A. & Hu, Y. (2021). An integrative investigation of sensory organ development and orientation behavior throughout the larval phase of a coral reef fish. Sci Rep11, 12377. https://doi.org/10.1038/s41598-021-91640-2
Mathew, P. & Bordoni, B. (2023). Embryology, Heart. In: StatPearls [Internet]. Treasure Island (FL): StatPearls Publishing; Available from: https://www.ncbi.nlm.nih.gov/books/NBK537313/
Mayer, C., & Joseph, K. S. (2013). Fetal growth: a review of terms, concepts and issues relevant to obstetrics. Ultrasound in obstetrics & gynecology : the official journal of the International Society of Ultrasound in Obstetrics and Gynecology, 41(2), 136–145. https://doi.org/10.1002/uog.11204
National Research Council (US) Committee on Technological Options to Improve the Nutritional Attributes of Animal Products. Designing Foods: Animal Product Options in the Marketplace. Washington (DC): National Academies Press (US); 1988. Muscle Cell Growth and Development. Available from: https://www.ncbi.nlm.nih.gov/books/NBK218163/
Nezwek, T.A., Varacallo, M. (2022). Physiology, Connective Tissue.. In: StatPearls [Internet]. Treasure Island (FL): StatPearls Publishing; Available from: https://www.ncbi.nlm.nih.gov/books/NBK542226/
Rey, R., Josso, N. & Racine, C. (2020). Sexual Differentiation. South Dartmouth (MA): MDText.com, Inc. Available from: https://www.ncbi.nlm.nih.gov/books/NBK279001/
Simon, A. K., Hollander, G. A., & McMichael, A. (2015). Evolution of the immune system in humans from infancy to old age. Proceedings. Biological sciences, 282(1821), 20143085. https://doi.org/10.1098/rspb.2014.3085
Udan, R. S., Culver, J. C., & Dickinson, M. E. (2013). Understanding vascular development. Wiley interdisciplinary reviews. Developmental biology, 2(3), 327–346. https://doi.org/10.1002/wdev.91
Velleman S. G. (2007). Muscle development in the embryo and hatchling. Poultry science, 86(5), 1050–1054. https://doi.org/10.1093/ps/86.5.1050
Wragg, J. W., Roos, L., Vucenovic, D., Cvetesic, N., Lenhard, B., & Müller, F. (2020). Embryonic tissue differentiation is characterized by transitions in cell cycle dynamic-associated core promoter regulation. Nucleic acids research, 48(15), 8374–8392. https://doi.org/10.1093/nar/gkaa563
Yan, X., Zhu, M. J., Dodson, M. V., & Du, M. (2013). Developmental programming of fetal skeletal muscle and adipose tissue development. Journal of genomics, 1, 29–38. https://doi.org/10.7150/jgen.3930
Comments