Medical Genetics: Chromosomal Aberrations
The intricate dance of life, orchestrated by the genetic code, relies heavily on the precise arrangement and integrity of chromosomes within each cell. These microscopic threads, composed of DNA and proteins, contain all the instructions that guide an organism's growth, development, and function. However, genetics can be disrupted by various factors, leading to what is known as chromosomal aberrations. These anomalies encompass a diverse range of genetic irregularities. They can manifest in various ways, from structural alterations in chromosomes to numerical imbalances. These aberrations can be the result of different factors, including genetic mutations, environmental exposures, or errors during the cell division process. These chromosomal aberrations can have profound effects on an individual's health, development, and overall well-being. In this article, the types, causes, consequences, and potential implications of chromosomal aberrations for medical research and treatment will be explored. In addition, the diseases or syndromes caused by chromosomal aberrations that have been studied more will be also presented, giving a comprehensive view of this topic.
Understanding Chromosomal Aberrations
In a healthy human being, the standard number of chromosomes in a cell is 46. These chromosomes are organized into 23 pairs, known as homologous pairs, making a total of 46 chromosomes. Each parent contributes one chromosome to each pair during reproduction, resulting in a diverse genetic makeup of their offspring. Homologous chromosomes have similar genetic information. These chromosomes are similar in terms of their size, shape, and the genes they carry, although they may have different alleles (gene variants). These chromosome pairs are crucial during sexual reproduction, as they undergo meiosis to produce gametes (sperm and egg cells), ensuring that the offspring inherits a diverse combination of genes from both parents. Non-homologous chromosomes, also known as heterologous chromosomes, are chromosomes that do not share significant structural or genetic similarity. They belong to different chromosome pairs and carry distinct sets of genes. In humans, for example, chromosome 1 is non-homologous to chromosome 2, and so on. These chromosomes play different roles in the genetic constitution of an individual.
Chromosomal aberrations are genetic anomalies that occur within an organism's chromosomes. Any deviation from the normal chromosomal makeup can result in a wide range of genetic disorders and diseases. Chromosomal aberrations can broadly be categorized into two types: numerical and structural aberrations. Any change in the total number of chromosomes in an individual, increasing or decreasing it, is considered a numerical aberration (Kirchmair, 1967) [Figure 1]. The most common numerical aberrations are the trisomies of any pair of chromosomes, where cells have an extra chromosome, and the monosomies, where cells lack a chromosome.

Structural aberrations, on the other hand, are represented by changes in the structure of chromosomes (Mantere et al., 2021) [Figure 2]. These changes can occur through various mechanisms, such as deletions, duplications, inversions, and translocations. Deletions involve the loss of a segment of a chromosome, which can be of any size, containing more or less genes, while duplications involve the presence of extra copies of a segment. In the case of duplications, the additional piece may get attached to the same chromosome or get inserted into another one, homologous or not, in the genome. Inversions are represented by the reversions of a portion of a chromosome that result in changes in the linear sequence of the genes in a chromosome, as if a piece of the chromosome breaks off, turns upside down, and reattaches again. This rearrangement usually does not result in a loss or gain of genetic material but can affect the order and orientation of genes. These structural anomalies can disrupt the normal functioning of genes, leading to a variety of genetic disorders.
Chromosomal aberrations can arise both as a result of the influence of environmental factors and inherent genetic errors. Exposure to certain environmental agents can increase the risk of chromosomal aberrations. Radiation, both ionizing (e.g., X-rays) and non-ionizing (e.g., UV radiation), can damage DNA and cause structural changes in chromosomes (Lefrançois et al., 1989). Chemical agents, such as certain carcinogens and mutagens, can also induce chromosomal abnormalities. Additionally, lifestyle factors like smoking and alcohol consumption during pregnancy can contribute to chromosomal aberrations in developing embryos (Farkas et al., 2021). Numeric aberrations in chromosomal content often arise as a result of errors during the cell division processes of gametocytes, namely eggs and spermatozoa. One of the prevalent anomalies in this context is known as non-disjunction, a phenomenon where chromosomes do not segregate properly during cell division, resulting in an unequal distribution of chromosomes among the resulting daughter cells. These numerical aberrations can occur spontaneously due to errors in the machinery of cell division, or they can be influenced by external factors such as environmental conditions or maternal age. Advanced maternal age, for instance, has been linked to an increased risk of non-disjunction events during the formation of eggs, contributing to a higher likelihood of chromosomal disorders in offspring.
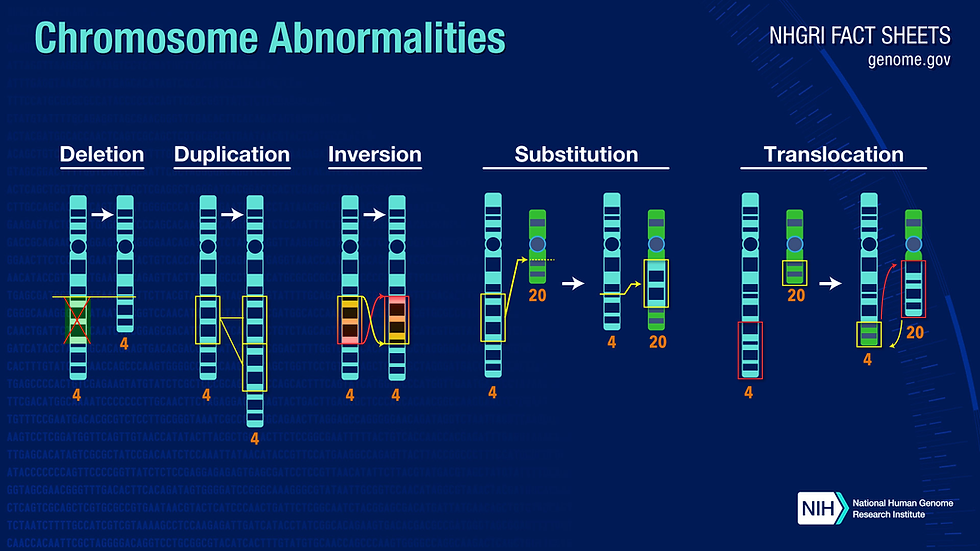
Normally, numeric aberrations cause nonviability of the affected fetus. However, there are some monosomies and trisomies that are viable, but cause severe syndromes with different symptoms, such as Down, Edwards, Patau, Turner, and Klinefelter, that will be deeply explored later in the article. Structural aberrations in genes tend to be less severe or life-threatening because they typically affect a smaller amount of genetic material compared to numerical aberrations. In contrast, structural aberrations are normally more associated with sterility and infertility, and many people can carry translocations and inversions without any symptoms until adulthood when they learn that they are sterile. However, there are also some syndromes and diseases that are associated with some specific structural aberrations, such as Edwards syndrome.
Diagnostic Techniques
In recent years, the field of genetics has experienced an extraordinary surge in the development of techniques for identifying chromosomal aberrations. These cutting-edge methods have revolutionized our ability to understand, diagnose, and manage genetic disorders, offering a spectrum of advantages in terms of precision, sensitivity, and specificity. This progress has profoundly impacted the work of clinicians, geneticists, and researchers, helping them delve deeper into the intricacies of the human genome and its implications for human health.
Karyotyping, one of the earliest chromosomal analysis methods, involves staining and photographing chromosomes during metaphase, a stage of cell division when chromosomes are condensed and aligned and can be visualized under a microscope. This technique allows for the visualization of the entire set of chromosomes by staining them with a combination of dyes that create distinct banding patterns in each pair of chromosomes. This technique allows the identification of numerical abnormalities (e.g., trisomies) and large structural changes such as deletions, duplications, translocations, and inversions, which can be detected by observing variations in the banding patterns or the positioning of chromosomes (Khazaei et al., 2022) [Figure 3].
Fluorescence In Situ Hybridization (FISH) is a technique that employs fluorescently labelled probes that specifically bind to the DNA. These probes are meticulously designed to be complementary to specific target sequences within the genome. Once applied to a biological sample, the probes seek out and precisely bind to their complementary sequences, enabling researchers to observe and analyze the location and distribution of these genetic elements with astonishing precision. It is especially useful for detecting small chromosomal abnormalities, such as deletions, duplications, and translocations (Bayani & Squire, 2004) [Figure 3]. A distinct advantage of FISH is its versatility in application, as it can be employed to visualize chromosomal content not only during metaphase, as seen in the karyotype, but also during interphase, the stage when cells are not actively dividing and when chromosomes cannot be seen. This capability enables researchers to study the spatial organization of genetic material within the context of a cell's nucleus in real-time.
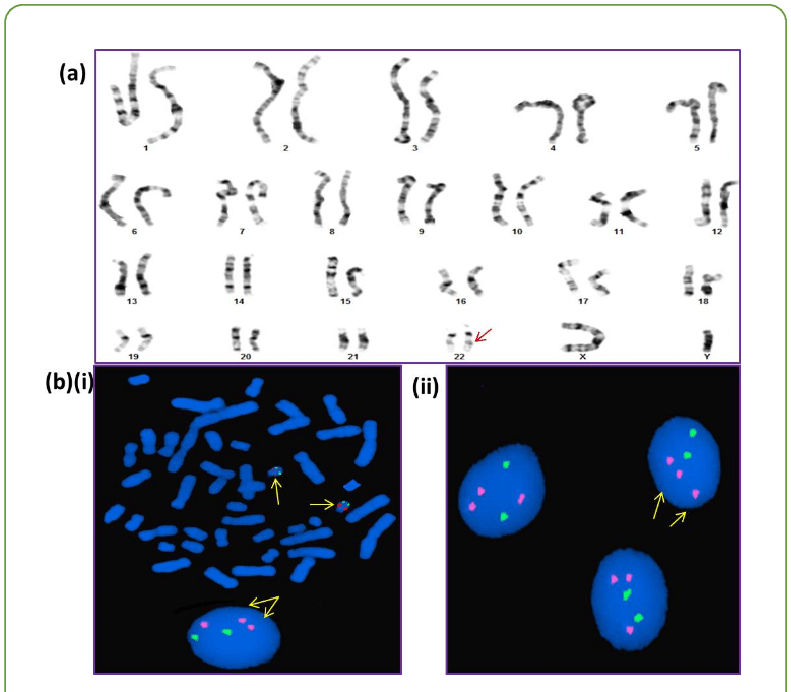
Comparative Genomic Hybridization (CGH) is a high-resolution technique that compares the DNA from an individual's cells with a reference DNA sample that is healthy. At its core, CGH involves a meticulous process of DNA comparison to unveil variations in DNA content across different samples. This technique is especially adept at detecting alterations in DNA dosage, such as numerical aberrations, deletions and duplications (Fiorentino, 2012). The procedure begins by extracting DNA from the patient samples and a reference DNA sample. These DNA samples are then differentially labelled with distinct fluorescent dyes. The individual's DNA is labelled with one colour (often green), while the reference DNA is labelled with another colour (often red). The marked DNA samples are subsequently mixed and allowed to hybridize or bind to complementary DNA sequences on a slide containing a comprehensive set of DNA fragments. During the hybridization process, any differences in DNA amounts between the individual's DNA and the reference DNA become apparent. If there is an imbalance in the amount of DNA, it is reflected in the relative intensity of the two different fluorescent colours at specific locations on the slide. This differential fluorescent signal reveals regions of the genome that have undergone deletions, duplications, or other structural changes.
Next-Generation Sequencing (NGS) has revolutionized genetic diagnostics by enabling rapid and comprehensive analysis of an individual's entire genome. One key application of NGS is Whole-Exome Sequencing (WES), which is designed to focus on the exome which refers to all the protein-coding regions of the genome. These exons comprise only a small fraction of the entire genome but harbour the majority of known disease-related mutations. WES offers a cost-effective approach to uncovering mutations responsible for a wide range of genetic disorders, including both rare and common diseases. On the other hand, Whole-Genome Sequencing (WGS) takes the analysis a step further by examining the entirety of an individual's genome, including not only the protein-coding regions but also the non-coding regions and repetitive sequences. WGS is more time-consuming and expensive than WES but it provides an all-encompassing view of an individual's genetic blueprint, enabling the identification of both coding and non-coding variants, structural rearrangements, and even epigenetic modifications (Petersen et al., 2017).
Non-Invasive Prenatal Testing (NIPT) stands as a revolutionary and transformative technique employed during pregnancy to gain crucial insights into the genetic health of the developing fetus. This method represents a significant advancement in prenatal screening, offering a safer and less invasive alternative to traditional diagnostic procedures such as amniocentesis, which holds the risk of injuring the fetus as it is based on the collection of amniotic liquid from inside the amniotic sac where the fetus is developing (Carbone et al., 2020). At the core of NIPT lies the analysis of cell-free DNA (cfDNA) derived from the placenta and present in the mother's bloodstream. This new approach has redefined prenatal genetic assessment by providing a wealth of information about the fetus's chromosomal makeup (Figure 4).
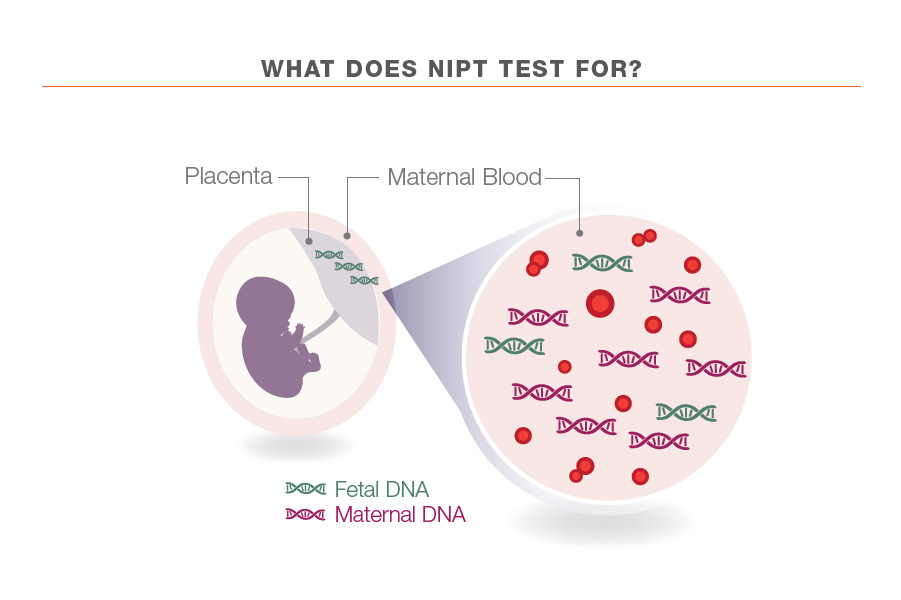
Advancements in diagnostic techniques have transformed the field of genetic diagnostics, presenting deep significance for patient care and medical research. However, while the progress in diagnostic techniques is remarkable, it also raises ethical considerations. Genetic information can have profound implications for an individual's life, family, and emotional well-being. Balancing the benefits of early diagnosis with the potential psychological impact is an ongoing challenge in the field of genetic counselling (Bredenoord et al., 2008).
Down Syndrome
Down syndrome holds a significant place in our understanding of human genetics, especially because of its impact on countless individuals across the globe. This condition was named after John Langdon Down, a pioneering physician who first documented its characteristics in 1866. Down syndrome is easily identifiable due to its unique combination of physical features and the developmental hurdles it presents. With a prevalence rate ranging from 1 in 800 to 1 in 1,000 births, Down syndrome ranks as the most frequently encountered genetic cause of developmental delays.
Down syndrome is also known as trisomy 21. It is a chromosomal disorder caused by the presence of an extra copy of chromosome 21 (Figure 5). This extra genetic material alters the course of development and gives rise to the characteristic features and challenges associated with the condition (Antonarakis et al., 2020). The overwhelming majority of cases of Down syndrome (about 95%) are caused by the normal trisomy of chromosome 21, leading to the physical and cognitive traits associated with Down syndrome. However, there are two rarer types of Down syndrome. In the case of translocation Down syndrome part of chromosome 21 breaks off and attaches to another chromosome, often to chromosome 14. While the total number of chromosomes is still 46, the presence of translocated genetic material from chromosome 21 can lead to Down syndrome characteristics (Jyothy et al., 2002). Mosaic Down syndrome is the other rarer case, it is a mixture of cells with the typical chromosomal arrangement and cells with trisomy 21. Individuals with mosaic Down syndrome may exhibit milder symptoms, as the extent of genetic disruption varies between cells (Papavassiliou et al., 2015).
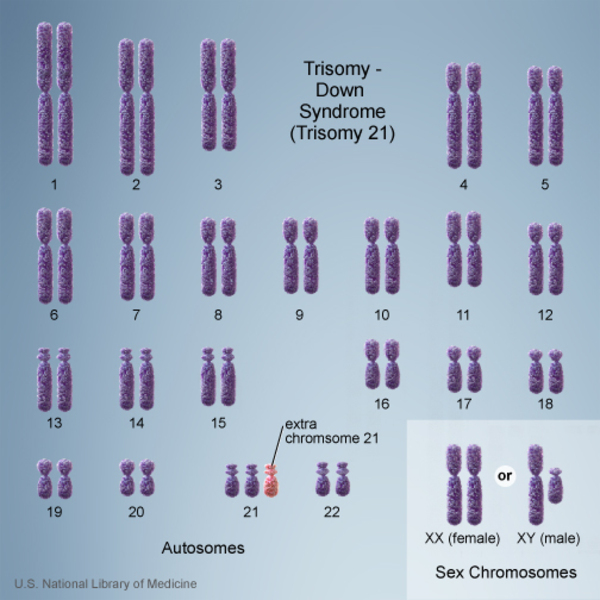
Individuals with Down syndrome exhibit a characteristic set of physical traits that, while not universal, are frequently observed and often serve as diagnostic markers for the condition. These traits may include almond-shaped eyes, a flat facial profile, brachycephaly (a specific skull shape characterized by a relatively short and broad head), a small nose, a short neck, and a single crease across the palm (Roizen & Patterson, 2003) [Figure 6]. Moreover, muscle tone is often reduced, leading to joint flexibility and delayed motor skills development. Cognitively, individuals with Down syndrome typically experience developmental delays, which can range from mild to moderate. Learning disabilities are common, but with early intervention and appropriate support, many can learn to communicate functionally and develop literacy skills. Speech and language development might also be delayed, though speech therapy can significantly aid in overcoming these challenges (Roizen & Patterson, 2003).
Down syndrome comes with an increased risk of other various medical conditions, although medical advances have improved the quality of life for individuals with the condition. Some common medical challenges include heart defects, gastrointestinal issues, respiratory infections, and thyroid problems among others.
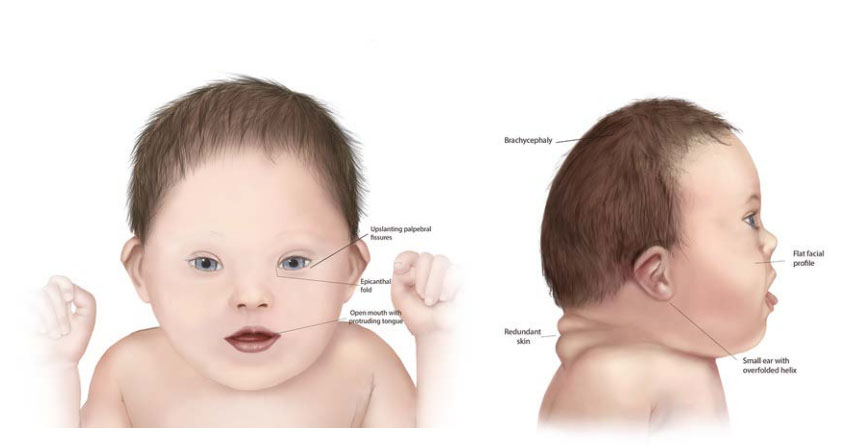
Early intervention plays a pivotal role in the supportive care of individuals with Down syndrome. Early childhood programs that include speech therapy, physical therapy, and occupational therapy, can help foster cognitive and motor development. Additionally, inclusive educational settings that provide individualized support can empower individuals with Down syndrome to achieve their full potential. As they transition into adulthood, individuals with Down syndrome can benefit from vocational training and job placement services that help them become active contributors to their communities. Social and emotional support networks also play a vital role in enhancing their well-being and sense of belonging (Agarwal Gupta & Kabra, 2014).
Edwards Syndrome
Edwards syndrome is also known as trisomy 18. The syndrome carries the name of physician John H. Edwards who was the first to describe it in 1960. Trisomy 18 is a rare chromosomal disorder with profound implications for affected individuals and their families, and it has an incidence of 1 in 2,000 to 6,000 births.
Edwards syndrome is characterized by the presence of an extra copy of chromosome 18 in the cells of the affected individual (Figure 7). This extra genetic material leads to a wide range of physical and developmental abnormalities, making Edwards syndrome a very challenging condition (Balasundaram & Avulakunta, 2023). About 95% of Edwards syndrome cases are the result of a random error during the formation of the egg or sperm cells. A rarer form of Edwards syndrome, called mosaic trisomy 18, occurs when only some of the cells in the body have the extra chromosome 18. This can result in milder symptoms, as the extent of genetic disruption varies among cells (Cereda & Carey, 2012).
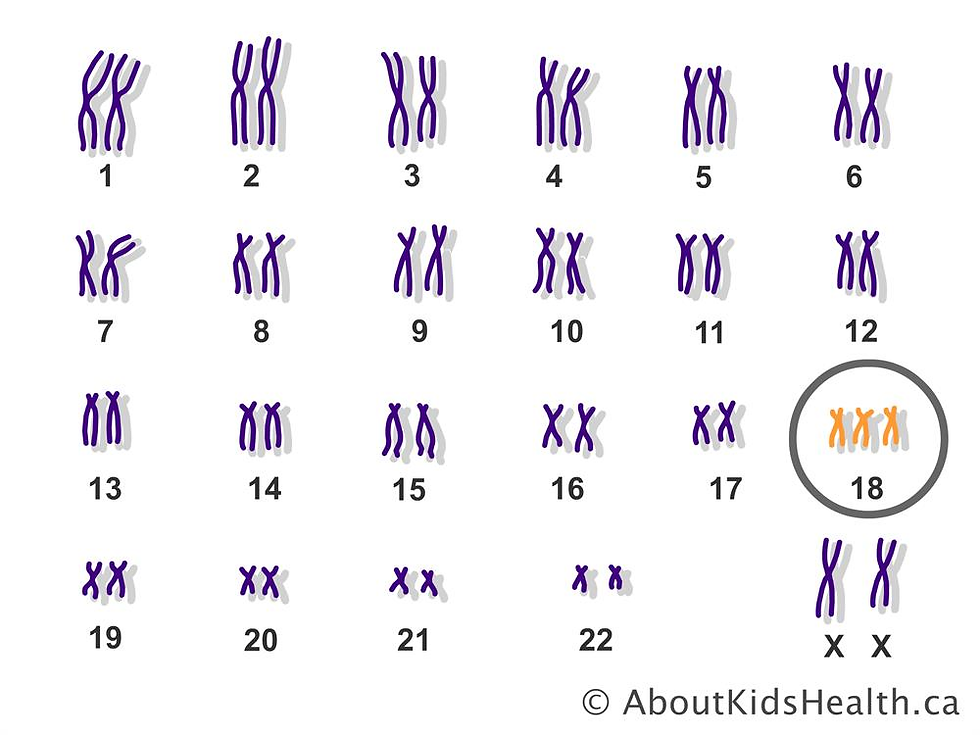
The symptoms of Edwards syndrome are both various and severe, affecting multiple systems of the body (Figure 8). These include craniofacial abnormalities, cardiac defects, growth, and developmental delays, hand and foot abnormalities, such as clenched fists with overlapping fingers and rocker-bottom feet, which can affect mobility and function, as well as internal organ anomalies, including kidney, gastrointestinal, and lung abnormalities, among others (Crawford & Dearmun, 2016). Managing Edwards syndrome involves a multidisciplinary approach, with the primary goal to provide supportive care and improve the quality of life of affected individuals.

Though Edwards syndrome remains a challenging condition with a high mortality rate, advancements in medical technology have extended the lifespan of some individuals with the disorder. Despite medical advancement, nowadays many individuals with this condition do not survive beyond infancy or early childhood, and many pass away during their first year of life. Prenatal screenings and diagnostic tests have improved the accuracy of detecting trisomy 18 during pregnancy, providing families with essential information for making informed decisions about care.
Patau Syndrome
Patau syndrome, also known as trisomy 13, was named after the physician Klaus Patau, who first described the syndrome in 1960. Trisomy 13 is a rare chromosomal disorder with profound implications for affected individuals and their families, and it has an incidence of 1 in 10,000 to 20,000 births. The extra genetic material of chromosome 13 can lead to a wide range of physical and developmental abnormalities, making Patau syndrome a challenging condition with a poor survival rate (Williams & Brady, 2023). A rarer form of Patau syndrome is a mosaic trisomy of chromosome 13, where milder symptoms may occur (Cammarata-Scalisi et al., 2019).
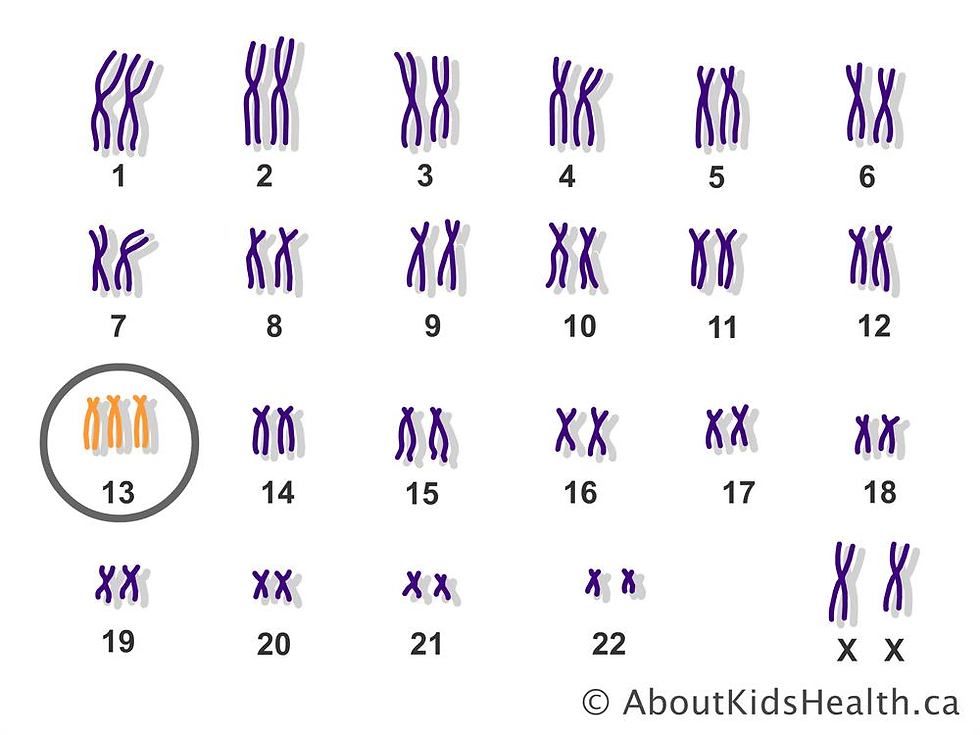
Patau syndrome is associated with a myriad of symptoms, many of which are severe and life-threatening. These include a cleft palate, where there is a gap or split in the roof of the mouth, a small head size which leads to significant intellectual disabilities, and ears that may be positioned on the head lower than usual (Figure 10). Furthermore, this syndrome can impact the development of internal organs, leading to conditions such as heart abnormalities and improper or missing development of certain internal organs. Since many of the symptoms of this syndrome can be life-threatening, nearly 80% of babies diagnosed with it do not survive past their first year (Pereira, 2023).

Turner Syndrome
Turner syndrome stands as a unique and intriguing thread. Discovered by Dr. Henry Turner in 1938, this genetic condition has captivated researchers, medical professionals, and families alike. Turner syndrome, also known as 45 X or monosomy X, is a chromosomal disorder that exclusively affects females. Typically, females inherit two X chromosomes (XX), but in Turner syndrome, one of the X chromosomes is missing or structurally altered (Steiner & Saenger, 2022) [Figure 11]. The primary cause of Turner syndrome is the complete or partial absence of one X chromosome. However, in some cases, mosaic Turner can also happen (Battin, 2003). It occurs in 1 in 2,000 to 3,000 live female births, being the most common sex chromosome abnormality in females (Steiner & Saenger, 2022).
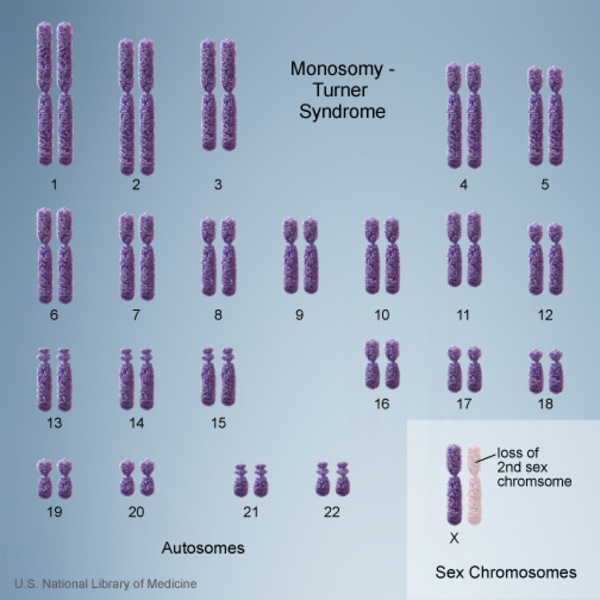
This genetic variation gives rise to a range of physical, developmental, and medical challenges. Symptoms and characteristics of Turner syndrome can vary widely, but some common signs and symptoms include short stature, webbed neck, low hairline, lymphedema (the swelling of body parts, typically in the arms or legs, due to the accumulation of lymphatic fluid), sterility and infertility, heart and kidney abnormalities, and learning and cognitive difficulties among others (Morgan, 2007) [Figure 12].
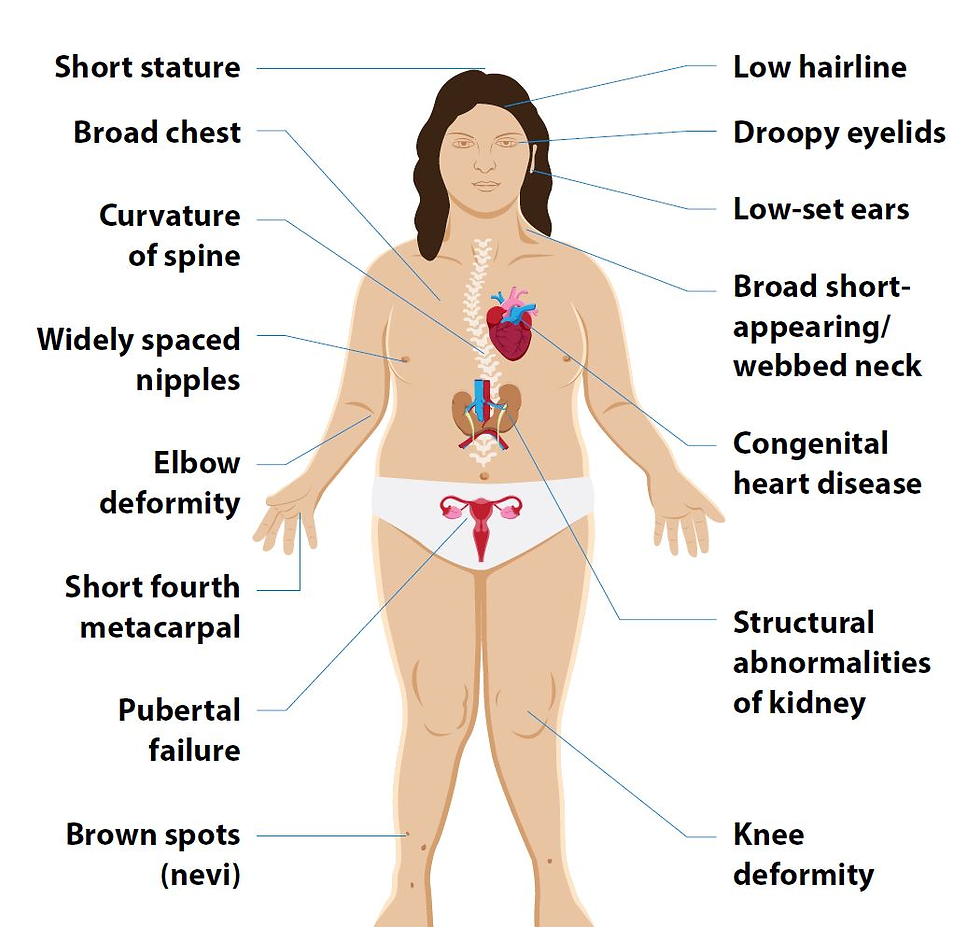
Klinefelter Syndrome
First described by Dr. Harry Klinefelter in 1942, this genetic condition challenges traditional notions of sex chromosome arrangements. Klinefelter syndrome, also known as XXY syndrome, is a chromosomal disorder that affects males. Typically, males inherit one X and one Y chromosome (XY), but in Klinefelter syndrome, an extra X chromosome is present, resulting in the chromosomal XXY configuration (Lanfranco et al., 2004) [Figure 13]. Rare variants of Klinefelter syndrome include cases such as XXYY, XXXY, and mosaic XXY/XY configurations, which can lead to different symptom severities (Hovnik et al., 2022). It affects 1 in 500 to 1,000 males, which makes it the most common chromosomal abnormality that causes male infertility (Lanfranco et al., 2004)

Klinefelter syndrome is a complex genetic condition that can manifest differently from one individual to another, making it a highly variable disorder. The principal characteristic is hypogonadism or the insufficient production of testosterone, the male sex hormone, by the testes. This hormone reduction leads to small testicles, reduced facial and body hair and incomplete development of secondary sexual characteristics such as broader shoulders and deeper voice. Affected individuals can also have fertility problems, learning and behavioural difficulties, osteoporosis, and reduced muscle mass (Figure 14). Hormone replacement therapy is a common approach to managing Klinefelter syndrome. This therapy involves the administration of exogenous testosterone to supplement the deficient levels, which can help alleviate many of the physical and psychological symptoms associated with the condition (Pizzocaro et al., 2020).

Williams Syndrome
First identified by the physician J.C.P. Williams in 1961, Williams syndrome, also known as Williams-Beuren syndrome (WS), is a genetic condition caused by a structural abnormality, specifically by a small deletion, a microdeletion, of a segment of chromosome 7. This microdeletion typically occurs sporadically and it is not inherited from parents. It results in the loss of approximately 26 to 28 genes. While the exact functions of these genes are still being studied, their absence or alteration leads to the unique combination of traits associated with Williams syndrome (Twite et al., 2019). It is a much rarer syndrome than the previously presented ones, affecting 1 in 7,5000 to 18,000 people.
Symptoms of Williams syndrome can manifest differently from one individual to another. However, one of the most recognizable features of Williams syndrome is the distinct facial appearance it imparts. This includes a broad forehead, a short nose with a flattened tip, and a wide mouth. These facial characteristics often make individuals with Williams syndrome easily distinguishable and can be one of the first indications of the condition (Figure 15). Apart from that, they can also have cardiovascular issues, developmental delay and intellectual disability (Stanley et al., 2021). Williams syndrome is also known for its unique personality profile. Individuals with this syndrome are often described as sociable, friendly, and highly empathetic. They tend to have excellent verbal and social skills, often striking up conversations with strangers easily.
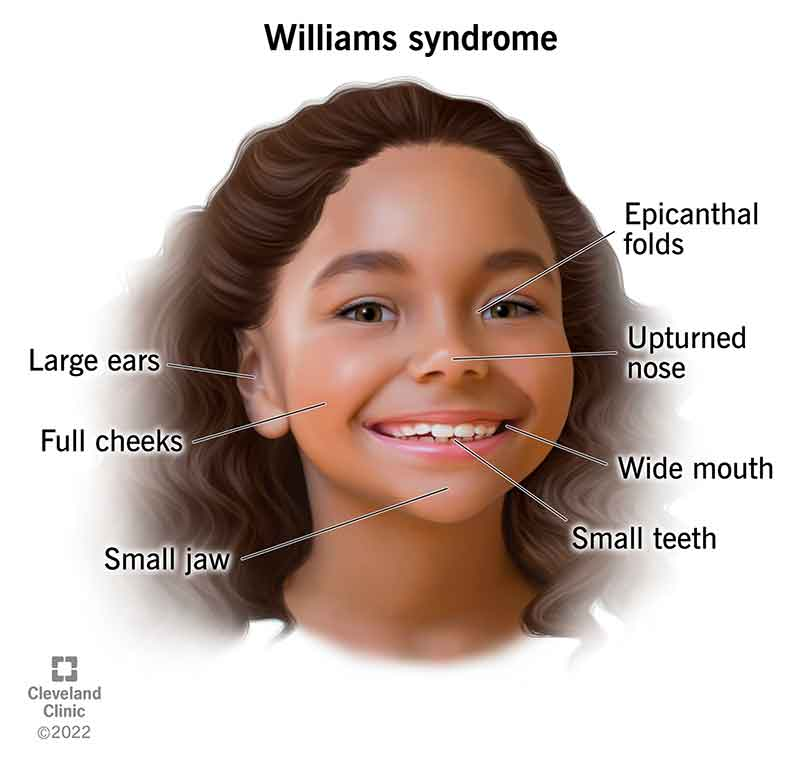
Conclusion
Chromosomal aberrations represent a multifaceted and diverse group of genetic anomalies that can have profound and lasting effects on an individual's life. These anomalies underscore the delicate and intricate balance that underlies genetic health, revealing both the fragility and resilience of the human genome. Within this realm, a wide spectrum of genetic anomalies exists, each with its unique characteristics and consequences. These anomalies are poignant reminders of the incredible complexity of our genetic makeup and the myriad ways in which it can be altered.
One of the most remarkable aspects of our evolving understanding of chromosomal aberrations is the significant progress made in diagnostic techniques. These advances have allowed for earlier and more accurate detection of these anomalies. With such capabilities, healthcare professionals can provide timely information to individuals and families, enabling them to make informed decisions about medical care and support. This precision has also paved the way for personalized treatment approaches tailored to an individual's unique genetic profile. As our comprehension of chromosomal aberrations continues to evolve, so do the possibilities in medical research and treatment too. Scientists and clinicians are constantly unravelling the complexities of these genetic irregularities. Their dedicated efforts offer hope for improved healthcare, a better quality of life for affected individuals, and a deeper appreciation for the intricate genetic tapestry that shapes us all.
Bibliographical References
Antonarakis, S.E., Skotko, B. G., Rafii, M. S., Strydom, A., Pape, S. E., Bianchi, D. W., Sherman, S. L., & Reeves, R. H. (2020). Down syndrome. Nature reviews. Disease primers 6(1), 9. https://doi.org/10.1038/s41572-019-0143-7
Balasundram, P., & Avulakunta, I. D. (2023, March 20). Edwards syndrome. In StatPearls. Treasure Island (FL): StatPearls Publishing. Retrieved on 29th, August 2023 from http://www.ncbi.nlm.nih.gov/books/nbk570597/
Battin, J. (2003). Turner syndrome and mosaicism. Bulletin de l’Academie nationale de medecine 187(2), 359–70. https://pubmed.ncbi.nlm.nih.gov/14556446/
Bayani, J., & Squire, J. A. (2004). Fluorescence in situ hybridization (FISH). Current protocols in cell biology Chapter 22: Unit 22.4. https://doi.org/10.1002/0471143030.cb2204s23
Bredenoord A.L., Pennings G., Smeets H.J., de Wert G. (2008). Dealing with uncertainties: ethics of prenatal diagnosis and preimplantation genetic diagnosis to prevent mitochondrial disorders. Human Reproductive Update 14(1):83-94. https://doi.org/10.1093/humupd/dmm037
Cammarata-Scalisi, F., Araque, D., Ramirez, R., Guaran, L., & Da Silva, G. (2019). Trisomy 13 mosaicism. Boletin medico del Hospital Infantil de Mexico 76(5), 246–50. https://doi.org/10.24875/bmhim.19000003
Carbone, L., Cariati, F., Sarno, L., Conforti, A., Bagnulo, F., Strina, I., Pastore, L., Maruotti, G. M., & Alviggi, C. (2020). Non-invasive prenatal testing: Current perspectives and future challenges. Genes 12(1). https://doi.org/10.3390/genes12010015
Cereda, A., & Carey, J. C. (2012). The trisomy 18 syndrome. Orphanet Journal of Rare Diseases 7(81). https://doi.org/10.1186/1750-1172-7-81
Crawford, D., & Dearmun, A. (2016). Edwards’ Syndrome. Nursing Children and Young People 28(10), 17. https://doi.org/10.7748/ncyp.28.10.17.s19
Farkas, G., Kocsis, Z. S., Szekely, G., Dobozi. M., Kenessey, I., Polgar, C., & Juranyi, Z. (2021). Smoking, chromosomal aberrations, and cancer incidence in healthy subjects. Mutation Research/ Genetic Toxicology and Environmental Mutagenesis 867, 503373. https://doi.org/10.1016/j.mrgentox.2021.503373
Fiorentino, F. (2012). Array comparative genomic hybridization: Its role in preimplantation genetic diagnosis. Current Opinion in Obstetrics & Gynecology 24(4), 203–9. https://doi.org/10.1097/gco.0b013e328355854d
Gupta, N. A., & Madhulika, K. (2014). Diagnosis and management of Down syndrome. Indian Journal of Pediatrics81(6), 560–67. https://doi.org/10.1007/s12098-013-1249-7
Hovnik, T., Zitnik, E., Avbelj Stefanija, M., Bertok, S., Sedej, K., Bancic Silva, V., Battelino, T., & Groselj, U. (2022). An adolescent boy with Klinefelter syndrome and 47 XXY/46 XX Mosaicism: Case report and review of literature. Genes 13(5). https://doi.org/10.3390/genes13050744
Jyothy, A., Rao, G. N. M., Kumar, K. S. D., Rao, V. B., Devi, B. U., & Reddy, P. P. (2002). Translocation Down syndrome. Indian Journal of Medical Sciences 56(3), 122–26. https://pubmed.ncbi.nlm.nih.gov/12508621/
Khazaei, E., Emrany, A., Tavassolipour, M., Mahjoubi, F., Ebrahimi, A., & Motahari, S. A. (2022). Automated analysis of karyotype images. Journal of Bioinformatics and Computational Biology 20(3), 2250011. https://doi.org/10.1142/s0219720022500111
Kirchmair, H. (1967). Numerical chromosome aberrations. Casopis lekaru ceskych 106(22), 600–603. https://pubmed.ncbi.nlm.nih.gov/5594453/
Lanfranco, F., Kamischke, A., Zitzmann, M., & Neischlag, E. (2004). Klinefelter’s syndrome. Lancet (London, England) 364(9430), 273–83. https://doi.org/10.1016/s0140-6736(04)16678-6
Lefrançois, D., Achkar, W. A., Couturier, J., Dutrillaux, A. M., Dutrillaux, B., Flüry-Herard, A., Gerbault-Seureau, M., Hoffschir, F., Lamoliatte, E., Lombard, M., Muleris, M., Prieur, M., Ricoul, M., Sabatier, L., & Viegas-Péquignot, E. (1989). Chromosomal aberrations induced by low-dose gamma-irradiation. Study of r-banded chromosomes of human lymphocytes. Mutation Research 212(2), 167–72. https://doi.org/10.1016/0027-5107(89)90068-7
Mantere, T., Neveling, K., Pabrel-Richard, C., Benoist, M., van der Zande, G., Kater-Baats, E., Baatout, I., van Beek, R., Yammine, T., Oorsprong, M., Hsoumi, F., Olde-Weghuis, D., Majdali, W., Vermeulen, S., Pauper, M., Lebbar, A., Stevens-Kroef, M., Sanlaville, D., Dupont, J. M., ... El Khattabi, L. (2021). Optical genome mapping enables constitutional chromosomal aberration detection. American Journal of Human Genetics 108(8), 1409–22. https://doi.org/10.1016/j.ajhg.2021.05.012
Gravholt, C. H., Viuff, M.H., Brun, S., Stochholm, K., Andersen, N. H. (2019). Turner syndrome: mechanisms and management. Nature Reviews Endocrinology 15 (3), 601-6014 https://doi.org/10.1038/s41574-019-0224-4
Papavassiliou, P., Charalsawadi, C., Rafferty, K., & Jackson-Cook, C. (2015). Mosaicism for trisomy 21: A review. American Journal of Medical Genetics. Part A 167(1), 26–39. https://doi.org/10.1002/ajmg.a.36861
Pereira, E. M. (2023). Trisomy 13. Pediatrics in Review 44(1), 53–54. https://doi.org/10.1542/pir.2022-005517
Petersen, B-S., Fredrich, B., Hoeppner, M. P., Ellinghaus, D., & Franke, A. (2017). Opportunities and challenges of whole-genome and -exome sequencing. BMC Genetics 18(1), 14. https://doi.org/10.1186/s12863-017-0479-5
Pizzocaro, A., Vena, W., Condorelli, R., Radicioni, A., Rastrelli, G., Pasquali, D., Selice, R., Ferlin, A., Foresta, C., Jannini, E. A., Maggi, M., Lenzi, A., Pivonello, R., Isidori, A. M., Garolla, A., Francavilla, S., Corona, G.King, & Klinefelter ItaliaN Group. (2020). Testosterone treatment in male patients with Klinefelter syndrome: A systematic review and meta-analysis. Journal of Endocrinological Investigation 43(12), 1675–87. https://doi.org/10.1007/s40618-020-01299-1
Roizen, N. J., & Patterson, D. (2003). Down’s syndrome. Lancet (London, England) 361(9365), 1281–89. https://doi.org/10.1016/s0140-6736(03)12987-x
Stanley, T. L., Leong, A., & Pober, B. R. (2021). Growth, body composition, and endocrine issues in Williams syndrome. Current Opinion in Endocrinology, Diabetes, and Obesity 28(1), 64–74. https://doi.org/10.1097/med.0000000000000588
Steiner, M., & Saenger, P. (2022). Turner syndrome: An update. Advances in Pediatrics 69(1), 177–202. https://doi.org/10.1016/j.yapd.2022.03.004
Twite, M. D., Stenquist, S., & Ing, R. J. (2019). Williams syndrome. Paediatric Anaesthesia 29(5), 483–90. https://doi.org/10.1111/pan.13620
Williams, G. M., & Brady, R. (2023, June 26). Patau syndrome. In StatPearls. Treasure Island (FL): StatPearls Publishing. Retrieved on 29th, August 2023 from http://www.ncbi.nlm.nih.gov/books/nbk538347/
Visual Sources
Cover Image: Chromosomal aberration: structural and numerical. [Image]. Plantlet. Retrieved on 29th, August, 2023 from https://plantlet.org/chromosomal-aberration-structural-and-numerical/
Figure 1: A karyotype of a woman which has an extra copy of the chromosome 23. [Image]. University of California San Francisco. Retrieved on 29th, August, 2023 from https://www.ucsfbenioffchildrens.org/medical-tests/karyotyping
Figure 2: The different types of structural aberrations. [Image]. National Human Genome Research Institute. Retrieved on 29th, August, 2023 from https://www.genome.gov/about-genomics/fact-sheets/Chromosome-Abnormalities-Fact-Sheet
Figure 3: Rewal, 2019. Karyotyping and Fluorescence In Situ Hybridization (FISH) depicting the duplication of a fragment of chromosome 22. [Image]. Journal of Biomedical Science. Retrieved on 29th, August, 2023 from https://www.researchgate.net/publication/337136465_Chromosomal_Microarray_Analysis_Uncovers_Pathogenic_Copy_Number_Variations_in_Unexplained_Neurodevelopmental_Disorders_and_Congenital_Anomalies
Figure 4: Non-invasive Prenatal Test (NiPT) screens for some of the most common genetic abnormalities using blood sample of the pregnant mother as it contains DNA from both mother and baby. [Image]. Quantum. Retrieved on 29th, August, 2023 from https://www.quantumdxs.com/patients/nipt
Figure 5: Down syndrome is caused by the trisomy of chromosome 21. [Image]. MedLine Plus. Retrieved on 29th, August, 2023 from https://medlineplus.gov/genetics/condition/down-syndrome/#causes
Figure 6: Common traits of Down syndrome. [Image]. Centers for Disease Control and Prevention. Retrieved on 29th, August, 2023 from https://www.cdc.gov/ncbddd/birthdefects/surveillancemanual/quick-reference-handbook/trisomy-21-down-syndrome.html
Figure 7:Edwards syndrome is caused by the trisomy of chromosome 18. [Image]. About Kids Health. Retrieved on 29th, August, 2023 from https://www.aboutkidshealth.ca/article?contentid=875&language=english
Figure 8: Common traits of Edwards syndrome. [Image]. Cleveland Clinic. Retrieved on 29th, August, 2023 from https://my.clevelandclinic.org/health/diseases/22172-edwards-syndrome
Figure 9: Patau syndrome is caused by the trisomy of chromosome 13. [Image]. About Kids Health. Retrieved on 29th, August, 2023 from https://www.aboutkidshealth.ca/article?contentid=876&language=english
Figure 10: Common traits of Patau syndrome. [Image]. Cleveland Clinic. Retrieved on 29th, August, 2023 from https://my.clevelandclinic.org/health/diseases/24647-trisomy-13-patau-syndrome
Figure 11: Turner syndrome is caused by the monosomy of chromosome X. [Image]. MedLine Plus. Retrieved on 29th, August, 2023 from https://medlineplus.gov/genetics/condition/turner-syndrome/
Figure 12: Common traits of Turner syndrome. [Image]. SingHealth. Retrieved on 29th, August, 2023 from https://www.singhealth.com.sg/patient-care/conditions-treatments/turner-syndrome
Figure 13: Klinefelter syndrome is caused by the presence of an extra chromosome X. [Image]. Bahceci. Retrieved on 30th, August, 2023 from https://bahceci.com/en/what-is-klinefelter-syndrome-what-are-the-types/
Figure 14: Common traits of Klinefelter syndrome. [Image]. SingHealth. Retrieved on 30th, August, 2023 from https://www.singhealth.com.sg/patient-care/conditions-treatments/klinefelter-syndrome
Figure 15: Common traits of Williams syndrome. [Image]. Cleveland Clinic. Retrieved on 30th, August, 2023 from https://my.clevelandclinic.org/health/diseases/15174-williams-syndrome
Comentários