Sniffing Science: From Humans to Dogs to Nano-noses
Our five senses - sight, hearing, touch, taste, and smell - are our connection to the outer world. The sense of smell (scent) is evolutionarily our oldest sense, yet it is also the most frequently disregarded. From single-celled bacteria to elephants, the top contenders in the list of species with the finest scent, all living organisms can detect odors, i.e., airborne chemicals in their surroundings. In fact, for most animal species, the primary window into the sensory world is the ability to recognize and adaptively respond to environmental chemical cues. Olfaction is, after all, the vertebrate analog of chemical sensing.
Making Sense of Senses
While most animals (and even our ancestors) rely on their sense of smell to seek food and water, find a partner, and communicate, modern humans navigate the world primarily through sight and hearing. As visually-oriented beings, we fail to comprehend the underlying significance of chemical sensitivity to life, or, in other words, the importance of our sense of smell (Hutmacher, 2019). It is both primitive and intricate, making it challenging to learn and even more difficult to understand how smell translates into our increasingly computerized world (Sarafoleanu et al., 2009). Our understanding of how our sense of smell influences the way we navigate the world lags far behind our grasp of hearing and vision. As we move safely through the world, we recognize friends (and foes) and identify objects through the power of sight, which allows the human brain to schematize the external environment (Hutmacher, 2019). Sound, on the other hand, not only enables people to communicate but also provides hints about the distances of objects (Middlebrooks & Green, 1991). While objects come in distinct shades, sizes, and forms, sounds come with different volumes, pitches, and tones, connecting us to a world in motion.
What about the sense of smell? It only takes a glass of milk and a piece of chocolate chip cookie to bring you back to your childhood. This is because the sense of smell is very deeply embedded in emotional memory (Herz & Engen, 1996). An odor is a chemical particle that enters the nose, attaches to olfactory receptor neurons and then travels to the brain's olfactory bulb, where it is converted into a format that the brain can interpret. Although these cells, like other neurons, extend a thicker fiber called an axon, olfactory axons are unique in that one end directly links to the brain, while the other projects to the outside environment (Rinaldi, 2007). This information is subsequently carried by brain cells to the amygdala, where emotions are decoded, and then to the neighboring hippocampus, where learning and memory formation happen (Kadohisa, 2013). This functional network enables a close relationship between memories, sensations, and odors. Only smells can reach the brain's emotions and memory regions in such a direct way.

Coming back to the crunchy cookie, try to pinpoint each scent you experience when eating it, without comparing it to any other smell. While this may seem like an easy task, you will almost surely fall short: there is more to odor than meets the eye. The main difficulty, however, stems from the fact that smells (which play a large part in what we call taste) are in themselves notoriously challenging to explain. This owes to the fact that the human race lacks an appropriate odor lexicon (Jönsson & Stevenson, 2013; Martina, 2022). The sense of smell is one of the most evocative senses, and probably the most difficult one to articulate.
Smelling in Stereo: A Dog’s Wet Nose
While humans rely primarily on sight to perceive their surroundings, spending more time deciphering visual data than olfactory information, dogs are the polar opposite: their remarkable sense of smell far surpasses that of humans. Although dogs use all of their senses to engage with the world, olfaction is their primary form of communication, not only conveying information about the current environment but also providing clues about the past, for example, the presence of a prey in the past (Siniscalchi et al., 2018). Dogs rely on the intensity of smells to keep track of time; while the weakest odors are the oldest, the strongest ones are also the newer. By distinguishing between new and old smells, dogs actually perceive events and substances across time intervals (Horowitz, 2016).
Dogs use this intricate web of distinct odors to create a three-dimensional picture of the environment over time, which is crucial when searching for food, identifying danger, or identifying suitable mates.(Kokocińska-Kusiak et al., 2021). Dog noses have up to 300 million olfactory receptors, compared to roughly 6 million in humans, and have a 40 times larger brain area devoted to scent analysis. Furthermore, dogs have neophilia, which makes them drawn to new and exciting odors (Phoenix Veterinary Center, 2023). In fact, the dog's odor detection capability has been claimed to be 10,000-100,000 times more than that of an ordinary human, with the canine's bottom limit of detection for volatile organic compounds being one part per trillion (Walker et al., 2006); that equates to half a teaspoon of salt dissolved in an Olympic-sized swimming pool.
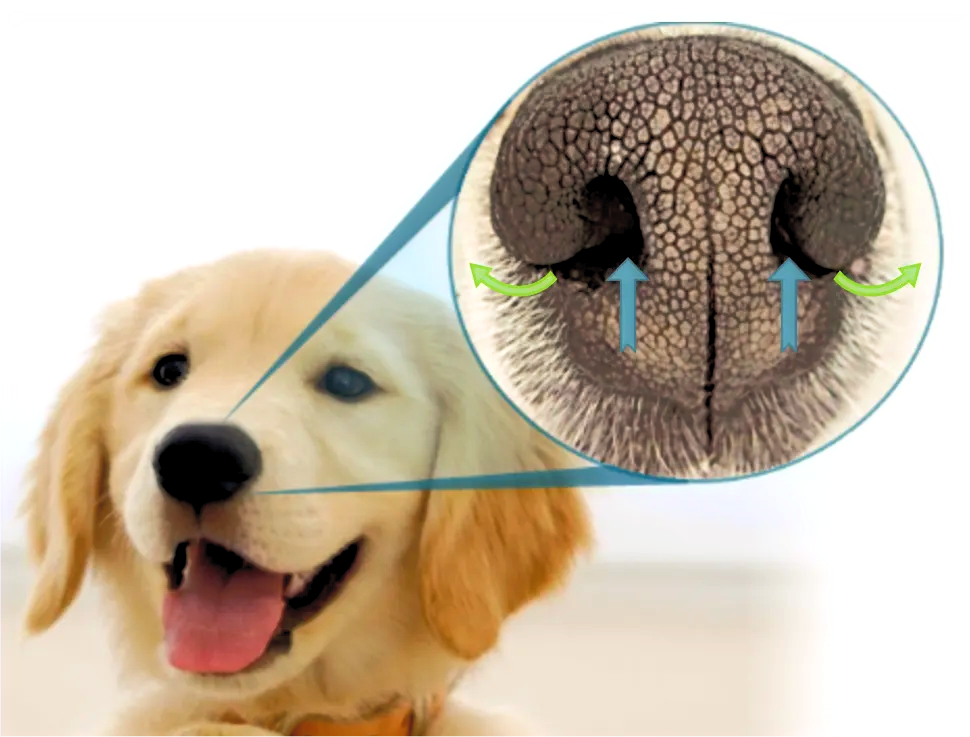
Unlike humans, dogs' noses are designed to allow air to flow in and out at the same time, creating continuous airflow. At the heart of this lies their ability to breathe in through their nostrils and out through slits on the side of their nose, in such a way that the smell of the incoming air is never diluted. When inhaling, up to 15% of the air that dogs inhale is diverted to the olfactory epithelium, where odor molecules are deposited and stored, preventing them from being exhaled (Craven et al., 2010). This membrane tissue is densely packed with olfactory receptors, which allow animals to perceive scents in minute quantities. By possessing large numbers of smell receptors, dogs can disentangle a wide variety of substances. The information about the scent molecules is subsequently conveyed to the brain's olfactory bulb, which is approximately 30 times bigger than that of humans (Otto, 2022). Furthermore, a dog’s nostril is smaller than the distance between them, giving dogs the ability to smell in stereo (Kavoi & Jameela, 2011). These traits combine to make a powerful nasal organ that conveys considerably more information than what human olfaction is capable of, decoding not just what, but also when, where, who, and even what is to come.
Master Sniffers: Dog Detectives on the Job
In the soft tissue of the nasal septum just above the palate lies the final piece of the dog's olfactory puzzle: the Jacobson's organ (also referred to as the vomeronasal organ). This organ serves as a secondary olfactory system, designed primarily for chemical communication and hence insensitive to common odors (Kokocińska-Kusiak et al., 2021). Rather, this organ responds to a variety of molecules that often have no discernible odor, for example pheromones, i.e., chemical compounds produced by the body that help build your olfactory footprint, but also volatile organic compounds (VOCs) that have been linked to various diseases including cancer (McGann, 2017). These are small molecules with a high vapor pressure, which makes them easily vaporizable to the gaseous state. The human body emits hundreds of VOCs whose components usually reflect a person's metabolic state. In fact, many diseases often result in a change in body odor which results in a disease-specific olfactory fingerprint. This olfactory fingerprint, also referred to as disease-specific VOCs, can be employed as non-invasive diagnostic olfactory biomarker not only for infectious diseases, metabolic diseases but also for genetic disorders, among others (Shirasu & Touhara, 2011).
The canine olfactory system thus consists of two major components, the major olfactory system (MOE) and the vomeronasal organ (VNO), which are physiologically autonomous and differ in architecture, function, and the molecules to which they respond. Additionally, signals sensed by MOE and VNO are sent to the brain through separate pathways (Swaney & Keverne, 2009). With this dual sniffing system, dogs are one-of-a-kind tracking masterpieces, widely used to detect explosives, narcotics, weapons, people and to a lesser extent, diseases. Although dogs are increasingly being employed in the detection of human illnesses, it took humans thousands of years to figure this out.
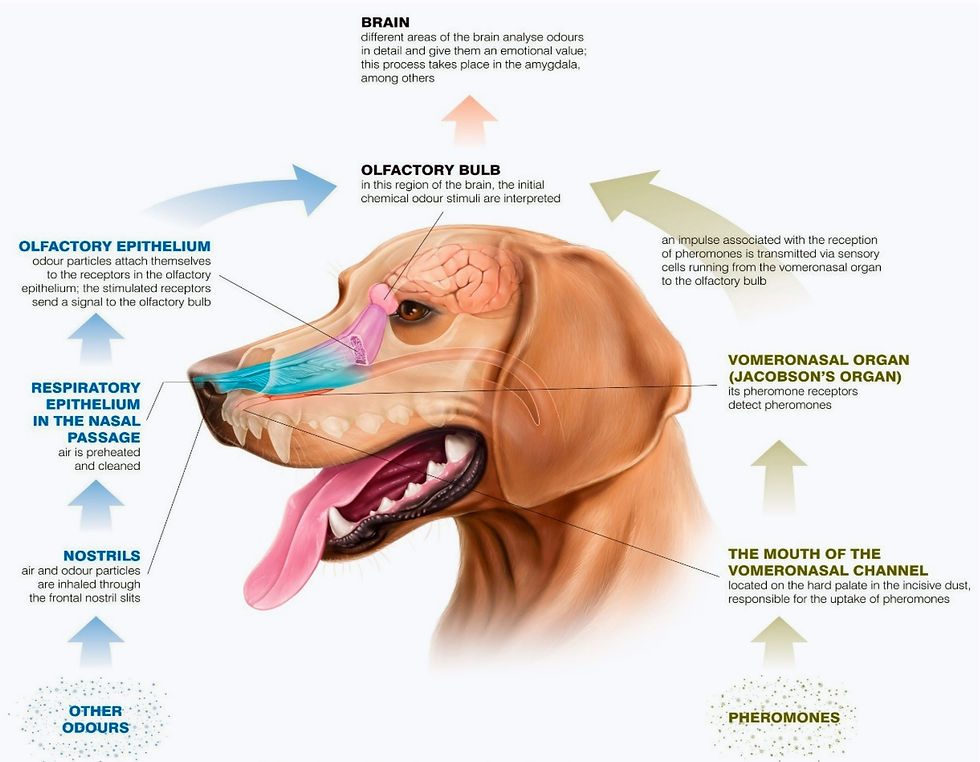
It was not until 1989 that doctors at Kings College Hospital in London first described a patient whose dog insisted on smelling a certain mole on her leg. This mole turned out to be an early-stage malignant melanoma (Williams & Pembroke, 1989). This case sparked the hypothesis that dogs may be powerful diagnostic tools and that cancer could be identified by scent. Numerous studies conducted all across the globe over the subsequent two decades evidenced that dogs can detect the scent of cancer. The remarkable discovery in 2004 by the British charity Medical Detection Dogs, which reported that dogs could diagnose bladder cancer in human urine, has sparked a huge surge in the training and use of dogs as a non-invasive diagnostic method (Willis et al., 2004).
British researchers have set a particularly high standard by training six dogs of different breeds to differentiate urine samples of people diagnosed with bladder cancer from samples of healthy controls or patients with other diseases. To test this, a carousel was constructed with eight equally spaced urine samples, one from a cancer patient and seven from individuals without cancer as a control. The overall diagnostic accuracy was 41% compared to 14% expected from chance alone (Willis et al., 2004). Interestingly, in a subsequent study, four dogs were subjected to rigorous training and double-blind testing, accurately identifying positive samples 64% of the time (Willis et al., 2011).
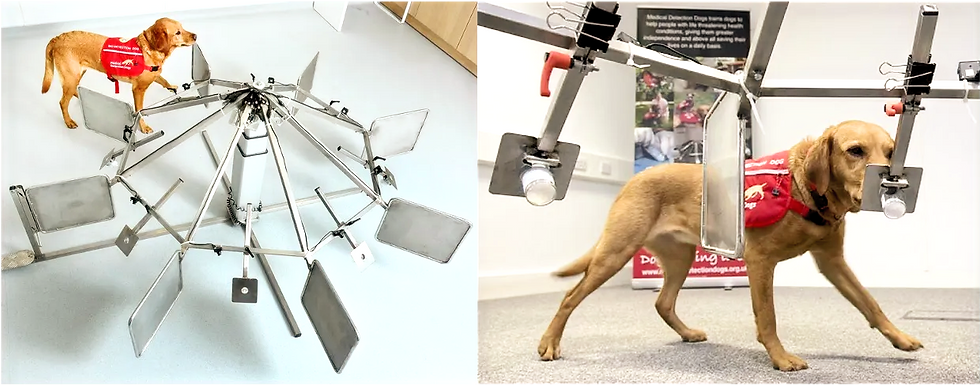
In an effort to establish whether dogs could be used as reliable disease detectors, a plethora of subsequent studies covering various cancer types were conducted around the world. Trained dogs have been shown to efficiently detect prostate (99.3% sensitivity) (Taverna et al., 2015), ovarian and lung (97.5% and 99% sensitivity respectively) (Horvath et al., 2008), and breast cancer (88% sensitivity) (McCulloch et al., 2006). Impressively, more recently, it has been revealed that dogs are capable of discerning a number of additional diseases, such as malaria (Guest et al., 2019), diabetes (Wells et al., 2008), and COVID-19 (Kantele et al., 2022). Dogs have proven to be the fastest and most precise scent-driven disease detectors ever studied.
Collaborative Searching: When a Dog’s Nose and AI Join Forces
Disease detection dogs are exceptionally important, yet training them on a broad scale is expensive, time-consuming, and currently impractical. These challenges have sparked an urge to develop devices that would automate the incredible olfactory abilities of dogs’ noses and brains. One promising direction is the development of nano-nose: a miniaturized detector system that rivals a dog’s nose. First introduced in 2021 as part of a collaborative project comprising multiple scientific institutes, this device contains mammalian olfactory receptors engineered to be 200 times more sensitive than a dog's nose, and hence capable of detecting tiny traces of a myriad of molecules or odors (Guest et al., 2021). But while dogs can infer certain patterns from scent, humans cannot glean the same information from chemical analysis, and neither can this device. One solution to the problem is to couple the device with a machine-learning process that can identify odor biomarkers of disease-carrying samples. By doing so, researchers were able to construct an artificial system with success rates matching those of dogs, with a score greater than 70%.
This ground-breaking discovery provides a solid foundation for future research to advance this technology to a clinically applicable level. However, to reliably determine the significant indicators of cancer, a much larger set of samples (approximately 5,000) must be tested. Such tests, however, are not cheap: each clinically tested and certified sample still costs around $1,000 (Guest, 2021).
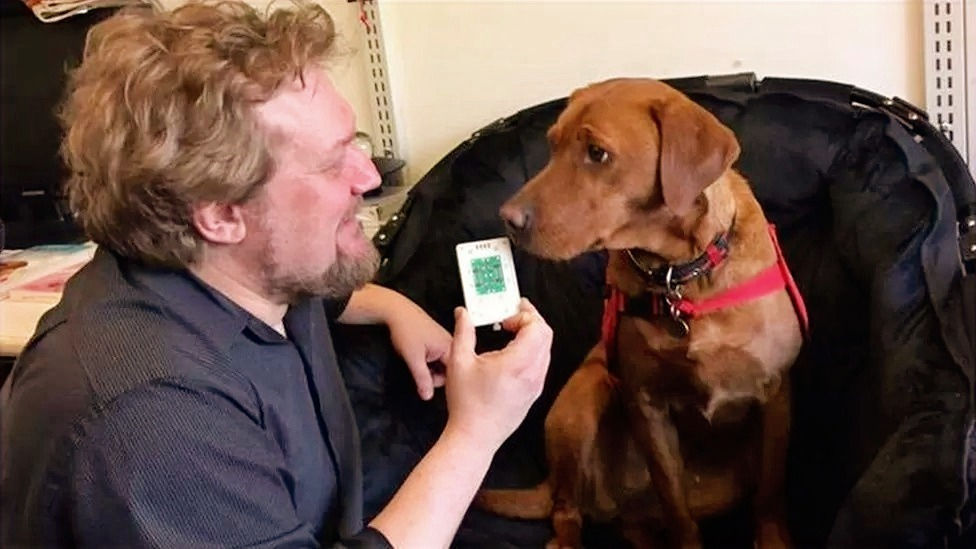
Whether it's detecting a fire or determining if food is safe, our nose is often the first to let us know something is wrong. Inspired by the outstanding canine scent, researchers are now designing advanced olfactory machines based on the intricate neuroscience that underpins our olfactory abilities. Electronic noses, or e-noses, are state-of-the-art devices designed to detect and react to VOCs, or in other words, specific chemicals in the air (odors). However, just like sniffer dogs, e-noses need to undergo extensive training to be effective. While sophisticated machine learning techniques can teach e-noses to recognize most odors dogs can smell, dogs still offer some advantages. Animals often understand which physiological and behavioral responses, such as fear or excitement, are most appropriate in the presence of an odor. However, in order to learn how to react and attribute odors to their primary sources, an e-nose must be instructed on data sets of VOC samples. Also, sensors are more sensitive to temperature and humidity than dogs and have a shorter lifespan. Given the processing power required and the multiple validation patterns the sensors must support, downsizing to the scale required for mobile or autonomous e-noses is another hurdle. Not to mention the challenge that legislation poses for the implementation of such devices on a large scale. Although a universal electronic nose capable of identifying any type of odor sample with high reliability and for all potential applications has yet to be developed, the future of e-noses holds promise. As technology progresses, new applications, especially for disease diagnosis, will emerge, allowing for faster analysis with greater accuracy and precision.
Bibliographical References
Craven, B. A., Paterson, E. G., & Settles, G. S. (2010). The fluid dynamics of canine olfaction: unique nasal airflow patterns as an explanation of macrosmia. Journal of The Royal Society Interface, 7(47), 933–943. https://doi.org/10.1098/rsif.2009.0490
Guest, C., Pinder, M., Doggett, M., Squires, C., Affara, M., Kandeh, B., Dewhirst, S., Morant, S. V., D’Alessandro, U., Logan, J. G., & Lindsay, S. W. (2019). Trained dogs identify people with malaria parasites by their odour. The Lancet Infectious Diseases, 19(6), 578–580. https://doi.org/10.1016/S1473-3099(19)30220-8
Guest, C., Harris, R., Sfanos, K. S., Shrestha, E., Partin, A. W., Trock, B., Mangold, L., Bader, R., Kozak, A., Mclean, S., Simons, J., Soule, H., Johnson, T., Lee, W.-Y., Gao, Q., Aziz, S., Stathatou, P. M., Thaler, S., Foster, S., & Mershin, A. (2021). Feasibility of integrating canine olfaction with chemical and microbial profiling of urine to detect lethal prostate cancer. PLOS ONE, 16(2), e0245530. https://doi.org/10.1371/journal.pone.0245530
Herz, R. S., & Engen, T. (1996). Odor memory: Review and analysis. Psychonomic Bulletin and Review, 3(3), 300–313. https://doi.org/10.3758/BF03210754
Horowitz, A. (2016). Being a Dog: Following the Dog into a World of Smell. Simon & Schuster.
Horvath, G., Järverud, G. af K., Järverud, S., & Horváth, I. (2008). Human ovarian carcinomas detected by specific odor. Integrative Cancer Therapies, 7(2), 76–80. https://doi.org/10.1177/1534735408319058
Hutmacher, F. (2019). Why Is There So Much More Research on Vision Than on Any Other Sensory Modality? Frontiers in Psychology, 10(October). https://doi.org/10.3389/fpsyg.2019.02246
Jönsson, F. U., & Stevenson, R. J. (2013). Odor knowledge, odor naming, and the “tip-of-the-nose” experience. Tip-of-the-Tongue States and Related Phenomena, 305–326. https://doi.org/10.1017/CBO9781139547383.014
Kadohisa, M. (2013). Effects of odor on emotion, with implications. Frontiers in Systems Neuroscience, 7(OCT), 1–6. https://doi.org/10.3389/fnsys.2013.00066
Kantele, A., Paajanen, J., Turunen, S., Pakkanen, S. H., Patjas, A., Itkonen, L., Heiskanen, E., Lappalainen, M., Desquilbet, L., Vapalahti, O., & Hielm-Björkman, A. (2022). Scent dogs in detection of COVID-19: triple-blinded randomised trial and operational real-life screening in airport setting. BMJ Global Health, 7(5). https://doi.org/10.1136/bmjgh-2021-008024
Kavoi, B. M., & Jameela, H. (2011). Comparative morphometry of the olfactory bulb, tract and stria in the human, dog and goat. International Journal of Morphology, 29(3), 939–946. https://doi.org/10.4067/S0717-95022011000300047
Kokocińska-Kusiak, A., Woszczyło, M., Zybala, M., Maciocha, J., Barłowska, K., & Dzięcioł, M. (2021). Canine olfaction: physiology, behavior, and possibilities for practical applications. Animals, 11(8), 2463. https://doi.org/10.3390/ani11082463
Martina, G. (2022). How we talk about smells. Mind and Language, August, 1–18. https://doi.org/10.1111/mila.12440
McCulloch, M., Jezierski, T., Broffman, M., Hubbard, A., Turner, K., & Janecki, T. (2006). Diagnostic accuracy of canine scent detection in early- and late-stage lung and breast cancers. Integrative Cancer Therapies, 5(1), 30–39. https://doi.org/10.1177/1534735405285096
McGann, J. P. (2017). Poor human olfaction is a nineteenth century myth. Science, 356(6338), 1–17. https://doi.org/10.1126/science.aam7263
Middlebrooks, J. C., & Green, D. M. (1991). Sound localization by human listeners. Annual Review of Psychology, 42(1), 135–159. https://doi.org/10.1146/annurev.ps.42.020191.001031
Otto, C. (2022). Sniffing out diseases. Nature, 606. https://media.nature.com/original/magazine-assets/d41586-022-01629-8/d41586-022-01629-8.pdf
Phoenix Veterinary Center. (2023). How Powerful Is a Dog’s Nose? https://phoenixvetcenter.com/blog/214731-how-powerful-is-a-dogs-nose
Rinaldi, A. (2007). The scent of life. The exquisite complexity of the sense of smell in animals and humans. EMBO Reports, 8(7), 629–633. https://doi.org/10.1038/sj.embor.7401029
Sarafoleanu, C., Mella, C., Georgescu, M., & Perederco, C. (2009). The importance of the olfactory sense in the human behavior and evolution. Journal of Medicine and Life, 2(2), 196–198.
Shirasu, M., & Touhara, K. (2011). The scent of disease: Volatile organic compounds of the human body related to disease and disorder. Journal of Biochemistry, 150(3), 257–266. https://doi.org/10.1093/jb/mvr090
Siniscalchi, M., d’Ingeo, S., Minunno, M., & Quaranta, A. (2018). Communication in dogs. Animals, 8(8). https://doi.org/10.3390/ani8080131
Swaney, W. T., & Keverne, E. B. (2009). The evolution of pheromonal communication. Behavioural Brain Research, 200(2), 239–247. https://doi.org/10.1016/j.bbr.2008.09.039
Taverna, G., Tidu, L., Grizzi, F., Torri, V., Mandressi, A., Sardella, P., La Torre, G., Cocciolone, G., Seveso, M., Giusti, G., Hurle, R., Santoro, A., & Graziotti, P. (2015). Olfactory system of highly trained dogs detects prostate cancer in urine samples. Journal of Urology, 193(4), 1382–1387. https://doi.org/10.1016/j.juro.2014.09.099
Walker, D. B., Walker, J. C., Cavnar, P. J., Taylor, J. L., Pickel, D. H., Hall, S. B., & Suarez, J. C. (2006). Naturalistic quantification of canine olfactory sensitivity. Applied Animal Behaviour Science, 97(2–4), 241–254. https://doi.org/10.1016/j.applanim.2005.07.009
Wells, D. L., Lawson, S. W., & Siriwardena, A. N. (2008). Canine responses to hypoglycemia in patients with type 1 diabetes. Journal of Alternative and Complementary Medicine, 14(10), 1235–1241. https://doi.org/10.1089/acm.2008.0288
Williams, H., & Pembroke, A. (1989). Sniffer dogs in the melanoma clinic? The Lancet, 333(8640), 734. https://doi.org/10.1016/S0140-6736(89)92257-5
Willis, C. M., Church, S. M., Guest, C. M., Cook, W. A., McCarthy, N., Bransbury, A. J., Church, M. R. T., & Church, J. C. T. (2004). Olfactory detection of human bladder cancer by dogs: proof of principle study. BMJ, 329(7468), 712. https://doi.org/10.1136/bmj.329.7468.712
Visual Sources
Cover image: Fowler, G. A. (2018). Aibo the robot dog will melt your heart with mechanical precision. The Washington Post [image]. https://www.washingtonpost.com/technology/2018/09/18/aibo-robot-dog-will-melt-your-heart-with-mechanical-precision/
Figure 1: Gillespie, C. (2021). This Is Why We Associate Memories So Strongly With Specific Smells. [image]. Mental Health News. https://www.verywellmind.com/why-do-we-associate-memories-so-strongly-with-specific-smells-5203963
Figure 2: Kim, C. (2022). Korean startup launching a nose-print identification for dogs, an alternate to the microchip embedding process. [image]. KoreaTechDesk. https://koreatechdesk.com/korean-startup-launching-a-nose-print-identification-for-dogs-an-alternate-to-the-microchip-embedding-process/
Figure 3: Kokocińska-Kusiak, A., Woszczyło, M., Zybala, M., Maciocha, J., Barłowska, K., & Dzięcioł, M. (2021). Canine Olfaction: Physiology, Behavior, and Possibilities for Practical Applications. Animals, 11(8), 2463. https://doi.org/10.3390/ani11082463
Figure 4: Medical Detection Dogs (2021). The Sniff. Issue 19. [image]. Dogs Saving Lives. https://www.medicaldetectiondogs.org.uk/sniff-magazine
Figure 5: Guest, C., Harris, R., Sfanos, K. S., Shrestha, E., Partin, A. W., Trock, B., Mangold, L., Bader, R., Kozak, A., Mclean, S., Simons, J., Soule, H., Johnson, T., Lee, W.-Y., Gao, Q., Aziz, S., Stathatou, P. M., Thaler, S., Foster, S., & Mershin, A. (2021). Feasibility of integrating canine olfaction with chemical and microbial profiling of urine to detect lethal prostate cancer. PLOS ONE, 16(2), e0245530. https://doi.org/10.1371/journal.pone.0245530
This article does a brilliant job of illustrating the incredible world of scent detection! The comparison between humans and dogs is fascinating, especially how dogs can outperform us in so many ways. I remember the time I had to train my dog to find hidden treats all over the house. It was amazing to see how quickly she adapted! If you want to dive deeper into this topic, tap here to learn more about our smaller brothers. The potential of nanonoses is an exciting frontier!